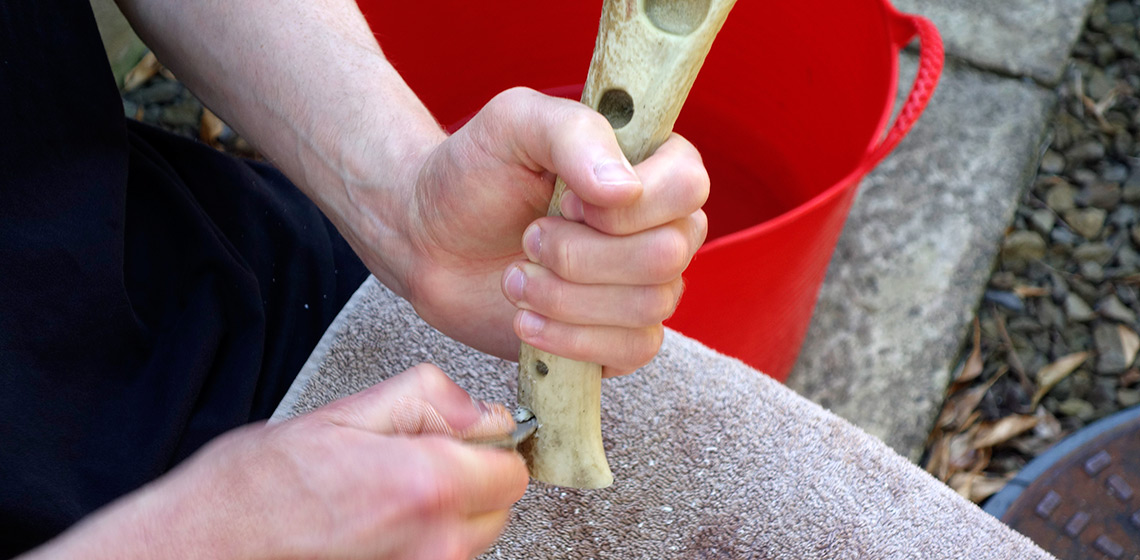
Perforations observed in artefacts, such as heavy tools, made from red deer antler indicate that Mesolithic people possessed various means for making holes in osseous materials. Nevertheless, prehistoric perforation technologies are relatively poorly understood. This study argues that a lack of systematic experimental-traceological work, compounded by the inadequacy of traditional modes of traceological data presentation, has deprived the literature of a robust empirical basis on which to interpret archaeological perforations. To help address this perceived gap in the literature, a series of experiments was undertaken in which different techniques were used to drill holes in red deer antler. The experimental material was then subjected to traceological analysis and photogrammetric modelling. Technologically diagnostic traces were identified by which two flint-based perforation techniques - hand boring and bow drilling - can be distinguished from one another. Relationships between the form of a flint tool and the morphology of the hole that it produces were also identified. Photogrammetry was evaluated as a method for the analysis and the presentation of osseous technological traces, and was found to have significant potential in this application.
Red deer antler 'heavy tools'
'Heavy tools' made from the antler of red deer are characteristic of Early Holocene hunter-gatherer groups throughout Europe north of the Mediterranean. These artefacts are identified by (i) a working edge created by an oblique truncation of the antler beam; and (ii) a perforation, roughly circular or oval in plan with a greatest width of around 2-3 cm, created through the full width of the antler beam to facilitate hafting (Smith, 1989, p.272; see Figure 1). Objects matching this description have been referred to in the literature as (T-)axes (for example, Riedel, Pohlmeyer and Von Rautenfeld, 2004), (hammer-)adzes (for example, Kabaciński, 2009), and mattocks (for example, Smith, 1989). 'Heavy tools' is used in the present study as a functionally agnostic term that captures all of these sub-types. Tools belonging to this broad category were utilised at various times in prehistory: direct radiocarbon dates from 17 British red deer antler heavy tools, for example, fell into two discrete clusters, one Mesolithic (circa 8000-4000 BC), the other Late Neolithic / Bronze Age (circa 2750-1200 BC; Tolan-Smith and Bonsall, 1998, p.254). They are, however especially important in Mesolithic material culture, with debates surrounding their manufacture, function, and social significance being crucial for our understanding of key issues in that period, such as the manner of transition from foraging to farming (Zvelebil, 1994) and the nature of intercultural contact and exchange (Czerniak, 2022).
A key technological feature of these artefacts is the perforation, the successful realisation of which is no mean feat given the large size of Early Holocene red deer in Europe. Yet the methods used to achieve these perforations are notoriously difficult to determine. First there are challenges presented by the archaeological material itself; any manufacturing traces left on the original perforation may be obliterated either by subsequent further working (for example, enlarging the hole by scraping with flint), by friction of the haft against the perforation walls during use, or by post-depositional processes (Czerniak, 2022, p.232; Orłowska, 2015, p.10). Then there are problems arising from the way in which the question has been treated academically. Interpretations of perforation technologies in the literature tend to fall into one of two categories: either they are offered as vague speculative hypotheses with no explicit empirical basis (perforations are created "probably with a bow drill and flint bit" (Smith, 1989, p.281) or "probably using some sort of drill" (Pratsch, 2011, p.90)); or the method of perforation is identified by virtue of traceological comparison with experimental material that is not published alongside the interpretation, making the latter unverifiable (for example, Kufel-Diakowska, 2011, pp.238-239; Kabaciński, et. al., 2008, p.286; Bergsvik and David, 2015, p.206; Broglio, et. al., 2004, p.58). Yet knowing how a perforation was made can be important: Czerniak (2022, pp.232-233) identifies the question of whether and how antler T-axes were drilled as key to understanding both the transmission of new technologies from farmers to hunter-gatherers and the social significance and symbolism of those objects in the north European Mesolithic-Neolithic transition. Therefore, these problems attending the technological interpretation of perforations are in need of a solution.
Characterising prehistoric perforation technologies: experiment and traceology
What is needed is a comprehensive published account of the technological traces associated with the different perforation techniques available in prehistory. Only with reference to such a resource could analysts arrive at empirically-grounded, transparent conclusions about how a given archaeological perforation was made. Two researchers have so far contributed to this enterprise. David (1999; 2008) documents three distinct drilling techniques, all of which she identifies traceologically in Mesolithic assemblages. 'Boring' involves the hand-rotation of a flint borer in an alternate semi-circular motion while applying downward pressure, resulting in a hole that is conical or (if drilled sequentially from opposing faces of the workpiece) biconical in cross-section (David, 2008, pp.83-85; see Figures 2a and 3a). This method leaves parallel to converging circular striations on the perforation walls (David, 1999, p.687). 'Drilling' involves the use of a hafted lithic drill tip operated with a bow, whereby an alternate back-and-forth motion is translated into a rotary one, resulting in a hole with a 'diabolo', 'double cylinder', or straight profile, and parallel, circular striations (David, 2008, pp.86-90; 1999, p.688; see Figures 2b and 3b). 'Coring' is the same as 'drilling' but uses a hollow, tubular drill bit of wood rather than a solid flint one, resulting in a cylindrical perforation, as observed in many antler heavy tools (David, 2008, pp.91-94; see Figures 2c and 3c).
More recently, Orłowska (2015) investigated a different set of three perforation techniques in an attempt to replicate experimentally the cylindrical perforations observed in red deer antler heavy tools from the Neolithic of Northern Europe. She used a more sophisticated fixed drilling apparatus to operate a drill shaft to which she attached in turn three types of drill bit: two core drill bits, of hollowed-out black elder shoot and pig metatarsal respectively, and a solid drill bit of flint. All three were found to produce holes of "almost ideally cylindrical shape" (Orłowska, 2015, p.9). Orłowska describes the traces observed on the perforation walls (See Figure 4): for the bone drill bit, "decomposing stacked linear traces of variable width, consisting of pits and polishing caused by friction of sand grains" (Orłowska, 2015, p.7); for the wood drill bit, "a different size of pitting and polishing, which creates distinct bundles of striae (of different bandwidth)" (Orłowska, 2015, p.8); and for the flint borer, striations that are "highly regular and evenly distributed, forming relatively wide linear traces situated one over another" (Orłowska, 2015, p.9).
These two studies represent valuable steps toward a robust empirical basis for identifying prehistoric perforation technologies. Yet they are not by themselves sufficient to this end. Their reporting of the crucial technological traces is generally quite thin on detail, comprising in each case only a few sentences and images, on the basis of which it is difficult to reliably interpret traces observed on archaeological perforations. To a large extent, the problem is inherent in the media used, not just in these studies but across the field, to present the results of traceological analyses: the qualitative, subjective character of written descriptions and the narrow, uncontextualised view afforded by two-dimensional images mean that publications necessarily fall well short of reproducing for the reader the experience of the analysts in examining first hand the traces on the actual physical object. Further work is needed to fill out and further refine these important first traceological descriptions of prehistoric perforation technologies.
Methodology: experiment, traceology, and photogrammetry
This conclusion provided the impetus for a series of experiments in which flint tools were used to perforate red deer antler. Rather than replicating specific lithic forms attested in the archaeological record, the decision was made to use the simplest possible form capable of doing the job; a simple flake, minimally retouched at the distal end so that it tapered to a central point. The aim of the experiments was to investigate the traceological consequences of manipulating specific technological variables. The first variable was the means by which the flint tool was set in motion, which was either by hand or using a bow drill. In hand boring, the point of the flint tool was pressed into the surface of the antler and, applying continuous downward pressure, rotated alternately clockwise and anticlockwise by hand until a hole was produced (See Figure 5). In bow drilling, the flint tool was hafted onto a drill shaft of wood that was then set in rotary motion using a bow, with the flint drill bit inserted into the antler (See Figure 6). These are essentially the first two of the three perforation techniques identified by David (2008, pp.83-90) and discussed above using her terms 'boring' and 'drilling'. It was considered important to revisit these already-documented techniques in order to test the repeatability of the reported results and to see if they could be further refined.
The second technological variable to be investigated was whether or not the antler was softened by soaking in water prior to working. It is well documented that preparing antler in this way can make it easier to work (MacGregor, 1985, pp.63-65; Langley and Wisher, 2019, p.11), and there is evidence that this was actually practised in prehistory (Elliott and Milner, 2010, p.81). Yet no one has asked whether this technological decision can be detected in the traces left by the method of perforation. In order to answer this question, half of the antler specimens were soaked in cool water for a minimum of five days prior to perforating.
Perforations were produced by hand boring and bow drilling on both dry and soaked antler. While other perforation techniques have been hypothesised in the literature, involving for example the use of solid or core drill bits of wood (for example, Riedel, Pohlmeyer and Von Rautenfeld, 2004, p.203) or bone (for example, Orłowska, 2015), these were considered outside the scope of the present study, which focused instead on the flint-based techniques more commonly referenced in archaeological interpretation. Prior to undertaking the experiments, the experimenter received tutorials on flint knapping and on constructing and using a bow drill before undertaking many hours of practice, during which a degree of competence was developed and different perforation techniques were trialled in order to refine the experimental design. In the final experiments, a perforation was regarded as complete when its depth reached half the width of the portion of antler in which it was created. Each completed perforation was examined both with the naked eye and using a 10 x 18 mm hand lens to identify macroscopic differences in (i) the morphology of the hole and (ii) the character of striations on its surfaces. Correlations between technological and traceological variables - that is, between differences in how a perforation was made and differences in the character of the traces thus produced - were identified and documented by way of photography and written descriptions. The limitations of these media have already been noted. Textual descriptions of traces are necessarily qualitative and subjective, and the terminology resorted to can therefore be notoriously esoteric (the description of polish distribution as resembling a 'melting snow field', for example) (Van Gijn, 2014, p.166). Photographs are equally problematic: the optics of close-range photography mean that images generally lack depth of field, and traces are therefore poorly contextualised, making fruitful comparison with physical archaeological objects difficult (Van Gijn, 2014, p.167).
In recognition of these limitations on the two traditional ways of presenting traceological data, a third was tested. Structure-from-Motion photogrammetry (hereafter simply 'photogrammetry') is a method whereby 3D digital models are created from real objects. The object is photographed from multiple overlapping viewpoints and these images fed into a computer program that calculates through phototriangulation the relative spatial locations of actual points on the object's surface, which can then be extrapolated to digitally reconstruct the surface of the whole object (Sapirstein, 2018, p.34). Photogrammetry has been tested as a way of capturing use-wear and technological traces on bronze swords (Molloy, et. al., 2016), stone tools (Zupancich, et. al., 2019), wood from shipwrecks (Eeckman, 2020), and butchered animal bone (Maté-Gonzalez, et. al., 2015; 2016; 2018). Its success in these applications gives reason to believe it might also be useful for documenting technological traces produced by perforating red deer antler. In order to test this supposition, photogrammetric models were generated of each perforation created in the course of the experiments. The digital models were evaluated in terms of their effectiveness at capturing the traceological features identified by the analysis as being technologically significant. The results of this evaluation are presented along with those of the experiments and traceological analysis below.
Results and discussion
Hand-bored and bow-drilled perforations were morphologically indistinguishable
Eight perforations were produced in the course of the experiments (See Table 1). They ranged in size from 16 to 23 mm maximum width and from 9 to 17 mm maximum depth, and took between 40 and 140 minutes of continuous work to complete. Each perforation conformed to one of two distinct morphological types. Type A (See Figure 7) is circular in plan and funnel-shaped in profile. Clearly, if this kind of perforation were replicated on opposing faces of the antler so that the two holes met in the middle, the result would be the biconical profile observed on many archaeological heavy tools (for example, Riedel, Pohlmeyer and Von Rautenfeld, 2004, p.203). Type B (See Figure 8) was more irregular, characterised by a rounded polygon shape in plan and a profile resembling an upside-down mushroom: the walls slope gently inwards in the upper portion before bulging abruptly outwards after penetrating a certain depth of spongy tissue, creating a slight overhang, with the walls of the perforation in this broader basal portion being rougher and messier. These two types were correlated with differences in the form of the flint tools that created them, as will be discussed in full below (See section entitled 'Lithic tool thickness determined perforation morphology'). Crucially, differences in perforation morphology were not found to be sensitive to differences in the way the flint tool was set in motion: when the same tool was used to produce two holes, one by hand boring, the other by bow drilling, those two holes were morphologically indistinguishable.
Perforation | 1 | 2 | 3 | 4 | 5 | 6 | 7 | 8 |
Technique | hand boring | hand boring | bow drilling | bow drilling | hand boring | hand boring | hand boring | hand boring |
Antler condition | dry | soaked | dry | soaked | dry | dry | soaked | soaked |
Maximum width (mm) | 18 | 16 | 19 | 23 | 21 | 23 | 19 | 24 |
Maximum depth (mm) | 11 | 9 | 12 | 11 | 13 | 16 | 11 | 17 |
Thickness of compact tissue (mm) | 3 | 6 | 3 | 7 | 3 | 4 | 3 | 3 |
Morphological type | A | A | A | A | B | B | B | B |
Tool width (mm) | 16 | 14 | 16 | 17 | 21 | 23 | 18 | 21 |
Tool thickness (mm) | 10 | 9 | 10 | 11 | 8 | 3 | 2 | 2 |
Tool width:thickness | 1,6 | 1,6 | 1,6 | 1,5 | 2,6 | 7,7 | 9,0 | 10,5 |
Total working time (minutes) | 50 | 80 | 40 | 80 | 75 | 140 | 40 | 55 |
Table 1. Descriptions of the eight perforations produced along with the tools that produced them. Note that ‘Tool width’ and ‘Tool thickness’ give the maximum width and thickness of the working part of the tool, that is the part that was actually in contact with the antler, since this is what is relevant in determining perforation morphology and traceology.
This result is in tension with earlier findings. David (2008, pp.85-87) reports that hand boring produces a biconical profile, consistent with the funnel shape described above, but characterises bow-drilled perforations as having a 'diabolo', 'double cylinder', or straight profile, and suggests that the two techniques may be distinguished on this basis (David, 2008, p.87; 1999, p.688; see Figure 9). However, the present analysis demonstrates that hand boring and bow drilling can in fact produce identical profiles, from which it follows that the two techniques cannot necessarily be distinguished by the shape of the perforation that they produce. David does recognise that tool form influences perforation morphology (her straight profile is produced by drilling with a double-backed point, for example (David, 2008, p.87), though she does not give detail on the tool forms associated with the other profiles she describes), so it may be that her morphological distinction between hand-bored and bow-drilled perforations is mediated by the tacit assumption that in prehistory different tool forms were used for hand boring on the one hand and bow drilling on the other. But if this is so, it is not clear what her justification is for this assumption. And in any case, the reported experiments prove that there are at least some tool forms that are equally suitable for use in both hand boring and bow drilling. Therefore, hand boring and bow drilling cannot be distinguished on the basis of perforation morphology alone.
Hand boring and bow drilling produced distinct patterns of striations
Hand-bored and bow-drilled perforations were readily distinguishable by the character of the striations observed on the surfaces, particularly of compact tissue, that had been abraded by the perforating lithic tool. Striations produced by bow drilling tended to be longer, horizontal, and parallel (See Figure 10), whereas those produced by hand boring tended to be shorter, oblique, and converging to intersecting (See Figure 11). In sum these differences amount to two quite distinct visual impressions, the striations on bow-drilled holes appearing very neat and regular, whereas those on hand-bored holes are rather messy. These differences are readily explained by the experiments. The mechanics of bow drilling are such that each single stroke of the bow, either back or forth, effects multiple full rotations of the drill bit in a single direction; when hand boring, each twist of the flint tool produces a rotation of at most around 180°. This explains the observed difference in the length of striations. Similarly, when bow drilling, an angle close to 90° is consistently maintained between the axis of perforation and the worked surface; but when hand boring, that angle is liable to vary slightly because the hand and wrist do not rotate perfectly about a fixed central axis. This explains the difference in the angle of striations.
No previous study has explicitly proposed a way of distinguishing traceological evidence between hand boring and bow drilling on the basis of striations. Elliott notes that hand-bored perforations often exhibit "fine, circular striations" (Elliott, 2012, p.53), and that bow-drilled perforations similarly have "small internal striations caused by the circular action of the shaft" (Elliott, 2012, p.54). He is not explicit as to whether hand-bored and bow-drilled striations are diagnostically different, but the similarity of the two descriptions suggests that they are not. On perforations made using a hafted flint drill bit and drilling apparatus, Orłowska recorded striations that were "highly regular and evenly distributed, forming relatively wide linear traces situated one over another" (Orłowska, 2015, p.9; see Figure 4c). These appear to be very similar to the parallel striations produced by bow drilling in the present experiments (See Figure 10). David (1999, pp.687-688) describes striations produced by hand boring as circular and parallel to converging, those made by bow drilling as circular and parallel. The distinction between convergent hand-bored striations and parallel bow-drilled ones is consistent with the results of the present study. But David does not report the other traceological distinctions here noted, such as that hand-bored striations are also shorter, more frequently intersect, and generally appear messier than bow-drilled ones. In fact, her (and Elliott's (2012, p.53)) description of hand-bored striations as circular appears to contradict the findings of the present study, according to which those striations are always shorter than the full circumference of the perforation walls, and therefore not circular. These findings help to fill out the to-date rather thin body of traceological data characterising hand boring and bow drilling, adding detail that variably compliments and contradicts previous contributions.
Striations tended to be deeper on soaked antler than on dry antler
Whether or not antler was softened by soaking prior to perforation was found to have no bearing on either the shape of the hole or the length and orientation of the striations produced. It did appear that striations on soaked antler tended to be deeper, and to that extent better defined, than those on dry antler. However, the relationship did not hold uniformly; it was not true that for any given soaked perforation its striations appeared to be on average deeper than those on any given dry perforation. Furthermore, another variable was identified that may have contributed to the variation in apparent depth of striations. All antler was collected, found either shed or attached to the skulls of dead animals, in the Scottish countryside, and as such the conditions to which it had been subjected and the length of time that had elapsed between deposition and use in the experiments was in every case unknown. It must therefore be conceded that there was an unknown and possibly significant degree of variation in the state of decay of antler specimens between experiments, that this may in turn have produced further variation in how the antler responded to soaking, and that the sum of these variations may have influenced to an unknown extent the observed differences in the depth of striations. (Incidentally, the same problem does not attend the above results regarding traceological identification of hand boring and bow drilling, since those relationships were observed consistently across the sample regardless of the material perforated.) Therefore, it must be concluded that in this instance control over nuisance variables was insufficient to determine whether the decision to soak antler prior to perforating can be detected traceologically, though the possibility of a correlation with the depth of striations may repay further study by way of more rigorous experiments.
Lithic tool thickness determined perforation morphology
While the experiments were designed only to investigate the traces associated with hand boring and bow drilling on dry and soaked antler, as it happened, they generated unplanned insights on another key variable in determining traces. This was the form of the flint tool used to make the perforation. In preliminary 'practice' experiments, which utilised smaller antler with thinner compact tissue from younger animals, a broad range of lithic implements was found to be effective: basically, any old flake would do, retouched or not. But in the final experiments, where perforations were made in larger antler (which more closely resembled those used to make Mesolithic heavy tools), it was found that lithic tools had to exhibit a certain prerequisite thickness in order for any of the techniques to operate smoothly (See Figure 12).
The property that was found to be crucial in determining the effectiveness of a lithic tool was the width:thickness ratio (W:T) of its working end (that is, the part of the tool where edges are actually in contact with the antler, abrading the walls and base of the perforation to enlarge it; see Table 1). When the tool used has W:T ≤ 2 (as when it has a sufficiently pronounced dorsal ridge), it follows that it must have three edges (the two lateral edges and dorsal ridge) in contact with the circumference of the perforation walls as it rotates (See Figures 13d and e). Three points of contact provide a stable base for a smooth, continuous rotary motion, enabling the tool to rotate about a fixed central axis that remains more or less perpendicular to the worked surface. The result is a hole conforming to morphological type A described above (See Figure 7): circular in plan, with a funnel-shaped profile reflecting the tapered shape of the tool that created it. The angle of slope of the perforation walls reflects the angles of the working edges of the tool: a steeply pointed tool will produce steeply sloping walls, a stubbier tool produces more gently sloping walls. This principle may explain David's proposed morphological distinction between bow-drilled and hand-bored perforations: for example, her conical profile indicates a tool with a straight-tapered form, whereas her diabolo profile suggests a tool with convex working edges (See Figure 9).
By contrast, when the tool has W:T > 2, it has only the two lateral edges in continuous contact with the perforation walls (See Figures 13a-c). This was found to make the tool inherently unstable when drilling or boring: the axis of perforation was not kept constant at 90° but tilted regularly, and the tool frequently became dislodged, especially when bow drilling. These problems began to manifest once the perforation reached a few millimetres in depth, and they increased as the depth increased. This resulted in a very consistent pattern in the course of the experiment: boring or drilling started smoothly, but once a certain depth was reached the tilting of the axis of perforation and consequent uneven abrasion around the perforation walls resulted in the formation of angles in the circumference of the hole, which became more pronounced as the experiment continued. These corners made it increasingly difficult to rotate the tool within the hole, and ultimately made further bow drilling impossible (though it was always possible, if laborious, to complete a perforation by hand boring). This consistent pattern of difficulties produced perforations conforming to morphological type B (See Figure 8): the formation of multiple corners resulted in a hole with a rounded polygon shape in plan, while the upside-down mushroom profile is thought to result from the erratic tilting of the axis of perforation, which caused the tip of the tool increasingly to gouge material laterally from the spongy tissue of the perforation walls as the hole deepened and the axis of perforation became increasingly unstable
On the basis of these observations it is hypothesised that any lithic tool with W:T ≤ 2 will produce a perforation that is circular in plan with a tapered profile (whose particular contours reflect the particular shape of the tool used), while a tool with W:T > 2 will produce a rounded polygon shape in plan and 'upside-down mushroom' profile. If true, how useful is this principle likely to be for interpreting archaeological perforations? On the one hand, it seems unlikely that any experienced prehistoric craftsperson would prefer thin flakes as blanks for perforating tools, since thick-flake tools are notably more effective (on average it took 78 minutes to complete a perforation by hand boring with a thin flake compared to 65 minutes with a thick one), and this fact is readily discoverable through minimal trial and error. It is however conceivable that such a craftsperson might resort to thin flakes in situations where lithic raw materials are scarce. After all, it is perfectly possible, if a little slower and more arduous, to produce a functional perforation in red deer antler by hand boring (though not by bow drilling) with a thin flake. Furthermore, the rounded polygon shape produced by thin flakes may actually deliver functional advantages over a circular hole, its angles perhaps helping to prevent the toolhead from spinning around in the haft.
So, are perforations exhibiting the morphology distinctive of thin-flake tools represented archaeologically? As already noted, the majority of heavy tools have holes that are circular or oval in plan with a biconical or cylindrical profile. But there are possible exceptions: perforations in antler T-axes from Dąbki, Poland, described as "oval and non-standardised" (Kabaciński and Winiarska-Kabacińska, 2023, p.5) with irregular internal surfaces, do appear to exhibit corners in the circumference of the hole, as well as the overhang distinctive of the 'upside-down mushroom' profile (See Figures 4:7, 5:2, and 5:4 in Kabaciński and Winiarska-Kabacińska, 2023, pp.5-6). An examination of the objects themselves would however be necessary to verify the apparent similarity.
It is worth recording one final observation made during the course of the experiments, this time regarding use-wear on the tools themselves. Tools made from thin-flake blanks (and to a much lesser degree those made from thick flakes) followed a consistent pattern of breakage through use: the force of the hard compact tissue at the perforation opening abrading against the lateral edges of the tool caused tiny chips to be periodically removed from those edges as the hole deepened, resulting in the formation of concave lateral working edges terminating in distinctive 'shoulders', whose distance from the tip of the tool corresponded to the final depth of the perforation (See Figure 14). One lithic perforator from Dąbki (See Figure 8:5 in Kabaciński and Winiarska-Kabacińska, 2023, p.9) exhibits precisely this form, indicating that it may have undergone the described pattern of breakage.
Limitations of the traceological analysis
The traceological analysis was subject to certain limitations, firstly concerning the nature of the dataset. Eight perforations were analysed, of which two were produced by bow drilling and six by hand boring (the asymmetry of the data being a result of the abovementioned failure to complete a perforation by bow drilling with thin-flake tools), while four perforations were made on soaked, four on dry antler, with four using thin-flake, four thick-flake tools. Given the modest sample size, there is considerable scope for further repetition of the experiments in order to fully establish the replicability of the results. Furthermore, while some training on the relevant techniques was received and a substantial amount of time spent practising prior to execution of the final experiments, it is acknowledged that the experimenter was nevertheless relatively inexperienced. Again, further repetitions of the experiments by others would be helpful to explore the possible effect of skill level as a variable influencing the results. Nevertheless, despite its small size, this dataset represents a significant advancement on the previously published experimental data on perforation production.
Further limitations relate not to the nature of the sample of material but to the way in which it was analysed. First, the scope of analysis was limited in terms of the kinds of traces that were considered, the focus being solely on the macroscopic character of striations and perforation morphology. Broadening the scope to consider other traceological features at different scales (for example, using low-power microscopy to examine polish on abraded surfaces) may have illuminated further relationships. Second, the traceological features that did fall within the scope of analysis were described only qualitatively. Observations such as that the striations on a given perforation were 'longer', 'deeper', or 'more often convergent' than those on another were not verified quantitatively (for example, by measuring the length, depth, and angle of striations), and therefore reflect a degree of subjective judgement. Finally, the experiments and traceological analysis were performed by the same individual, meaning that analysis was undertaken in full knowledge of precisely how each perforation had been made. This awareness may plausibly have primed the analyst to recognise patterns where patterns were expected, thus creating biases. Analysis of blind samples is therefore required in order to test whether the identified relationships can be used to reliably infer perforation technique from character of traces when the former is unknown to the analyst. In light of these limitations on the analysis, further study is required to test the robustness of the results here reported. Recommendations for such are elaborated in the conclusion.
3D models were useful for studying perforation morphology
3D models of all completed perforations were evaluated in terms of how effectively they captured the traceological and morphological features identified by the foregoing analysis as being technologically significant. As expected, striations were not represented in the structure of the models themselves (See Figure 15a); the density and accuracy of points were simply not sufficient to capture such fine details of surface topography. Striations were represented on the textured models, which are generated by mapping the 2D colour detail of the original photographs onto the surface of the 3D models. But due to insufficient depth of field in the original images, even the textured models depicted striations only in low-resolution, permitting at best a vague impression of the general character of striations to be gained. While this was sufficient to facilitate discernment of neater bow-drilled striations from messier hand-bored ones using the models (See Figures 15b and c), photography was found to communicate the distinction more clearly: by manipulating the position of the light source relative to the captured surface, one can compose an image so that the differential reflection of light makes even some very fine striations more clearly visible (See for example Figure 10b). But this technique can be used to illustrate clearly the traces on only a small area of the object's surface in any single image. An advantage of the textured 3D models in this respect is that they can be rotated to view striations on different surfaces dynamically in relation to one another, allowing for a more holistic examination than is afforded by a snapshot from a fixed perspective. It may be possible, through careful lighting of the subject during image capture and subsequent application of the 'focus stacking' technique (See for example Angheluță and Rădvan, 2019), to produce textured models that depict striations in good definition on all surfaces, thus combining the advantages of photogrammetry with those of traditional photography; this approach is recommended for future studies.
The models were more useful for examining perforation morphology. Constraints on processing power and available hardware (images were shot freehand rather than using a tripod and turntable) meant that the models were rather low-resolution in terms of their numbers of points, faces, and vertices, and therefore representation of surface shape was relatively coarse. Nevertheless, each of the models is adequate to facilitate recognition of either the circular or rounded polygon shape distinctive of thick- and thin-flake perforation respectively (Figures 16a and b). For this purpose, the 3D models are at least as effective as traditional photography. Indeed, in cases where the characteristic corners produced by thin flakes are more subtle, the dynamic nature of the models gives them an edge over static images, since often the best single viewpoint from which to see a certain corner renders the remaining corners invisible, and so in order to get a sense for the whole shape it is preferable to have freedom of movement between viewpoints.
The models are also very useful for interrogating profiles: on every model the characteristic funnel or inverted mushroom profile was easily recognisable. Particularly valuable was the ability to view the perforation apparently inside-out, from within the antler itself, as though it were a solid object rather than a negative feature (See Figures 16c and d). This was found to facilitate visualisation of the perforation's 3D shape in a way that even an examination of the physical object itself could not match. It was also possible to create a half-section of the perforation by slicing the model vertically in two (See Figures 16e and f), another useful way of inspecting profiles virtually that would be impractical to replicate on the physical object. For viewing profiles 3D models are vastly superior to traditional alternatives: the practical challenges posed by such small holes make it impossible to photograph, and difficult even to accurately draw, the profile in full. Thus, the models proved very useful for the analysis and presentation of morphologies, despite their relatively low-resolution reconstruction of surface shape.
Conclusions
The introduction to the present study argued that the literature was in need of a more robust empirical basis for the identification of prehistoric perforation technologies, such as those utilised to make red deer antler heavy tools. The results presented contribute towards achieving this goal in two ways: first, they build on previously published experimental-traceological studies to fill out and refine our understanding of archaeological perforation techniques and their traceological signatures; second, they point the way towards further refinement of this understanding by identifying key techno-traceological relationships for further investigation. This article will close by summarising the main findings along with some questions that these pose for future research.
- Perforations produced by hand boring and bow drilling were morphologically indistinguishable. This result contradicted David's (2008, pp.85-7) suggestion that hand-bored and bow-drilled perforations can be distinguished by their different profiles. An interesting question is, therefore, what variables are responsible for this discrepancy in results? Under what circumstances do hand boring and bow drilling produce perforations with distinct morphologies?
- Hand boring and bow drilling produced distinct patterns of striations. Striations produced by bow drilling were perceived to be longer, horizontal, and parallel, whereas those produced by hand boring were shorter, oblique, and converging to intersecting. Can this observed relationship between perforation technique and character of striations be verified quantitatively and described in terms of measured lengths and angles of striations, such that it could be used to reliably infer the method of perforation for blind samples of material? And can a more sophisticated traceological examination, for example using different grades of microscopy, identify further techno-traceological relationships to strengthen the empirical basis for such inferences?
- Striations tended to be deeper on soaked antler than on dry antler. Can further experiments, crucially exercising greater control over nuisance variables such as condition of antler, support or disprove the hypothesis that, all else being equal, striations produced by the same tool using the same technique are deeper on soaked than on dry antler? And again, can this relationship, suggested here on the basis of qualitative observation, be proven quantitatively, in terms of a numerical difference in the average depth of striations?
- Lithic tool thickness determined perforation morphology. A specific relationship was postulated: any tool with W:T ≤ 2 will produce a perforation that is circular in plan with a tapered profile, while a tool with W:T > 2 will produce a rounded polygon shape in plan and 'upside-down mushroom' profile. Can this hypothesis be supported or refined by further experiments? Among the limited sample studied, tool form was found to be not only an important variable but the only important variable in determining perforation morphology (with a single tool producing identical morphologies by hand boring and bow drilling on dry and soaked antler). Therefore, future studies should ask what other specific relationships can be identified between tool form and perforation morphology. What other properties of tools (besides W:T) are salient in predicting the shape of the hole they will produce?
- 3D models were useful for studying perforation morphology. The former allow the three-dimensional shape of a perforation to be quickly and intuitively apprehended by the viewer in a way that cannot be matched by photographs. By allowing the perforation to be viewed inside-out and in half-section, the 3D model facilitates novel and informative perspectives on morphology that even a physical encounter with the original object cannot provide. It is therefore concluded that photogrammetry has considerable potential as a tool for the sharing of traceological data on perforations. If 3D models were to appear in publications alongside the traditional written descriptions, photographs, and drawings of traces, this would certainly enhance the ability of readers to compare technologically salient features of archaeological perforations with experimental reference material in order to more reliably interpret the former. Future studies should aim to refine methodologies for the construction of 3D models in order to maximise their utility in this application. In particular, ways of achieving better visualisation of striations should be sought, perhaps utilising macrophotogrammetry to capture finer detail in the object's surface topography (for example, Mate-Gonzalez, et. al., 2015; 2016; 2018).
Bibliography
Angheluță, L. and Rădvan, R., 2019. Macro photogrammetry for the damage assessment of artwork painted surfaces. The International Archives of Photogrammetry, Remote Sensing and Spatial Information Sciences, 42, pp.101-107.
Bergsvik, K. and David, É., 2015. Crafting bone tools in Mesolithic Norway: A regional eastern-related know-how. European Journal of Archaeology, 18(2), pp.190-221.
Broglio, A., Cilli, C., Giacobini, G., Guerreschi, A., Malerba, G. and Villa, G., 2004. Typological and technological study of prehistoric implements in animal hard tissues. Collegium antropologicum, 28(1), pp.55-61.
Czerniak, L., 2022. T-shaped antler axes and their role in building exchange networks in the southwestern Baltic zone in the 5th millennium cal BC. In: M. Grygiel and P. Obst, eds. 2022. Walking among ancient trees: Studies in honour of Ryszard Grygiel and Peter Bogucki on the 45th anniversary of their research collaboration. Kraków: Profil-Archeo Publishing House. pp.222-240.
David, E., 2008. Principes de l'étude technologique et critères de diagnose des techniques mésolithiques. Séminaire de technologie osseuse. Université Paris X Nanterre.
David, E., 1999. L'industrie en matières dures animals du Mésolithique ancient et moyen en Europe du Nord. Contribution de l'analyse technologique à la definition du Maglemosien. Ph. D. Université Nanterre-Paris X.
Eeckman, A., 2020. Studying woodworking technology on ancient shipwrecks through digital photography and photogrammetry. In: Honour Frost Foundation, MAGS 2019, Maritime Archaeology Graduate Symposium. Southampton, United Kingdom, 29-31 March 2019. London: Honour Frost Foundation.
Elliott, B., 2012. Antlerworking Practices in Mesolithic Britain. Ph. D. University of York.
Elliot, B., 2015. Facing the Chop: Redefining British Antler Mattocks to Consider Larger-scale Maritime Networks in the Early Fifth Millennium Cal BC. European Journal of Archaeology, 18(2), pp.222-244.
Elliott, B. and Milner, N., 2010. Making a Point: a Critical Review of the Barbed Point Manufacturing Process Practised at Star Carr. Proceedings of the Prehistoric Society, 76, pp.75-94.
Kabaciński, J., 2009. Quarrying the antler adzes-a new Mesolithic site of the Boreal period at Krzyż Wielkopolski, western Poland. Quartär-Internationales Jahrbuch zur Erforschung des Eiszeitalters und der Steinzeit, 56, pp.119-130.
Kabaciński, J., David, E., Makowiecki, D., Schild, R., Sobkowiak-Tabaka, I. and Winiarska-Kabacińska, M., 2008. 'Stanowisko mezolityczne z okresu borealnego w Krzyżu Wielkopolskim'. Archeologia Polski, 53(2), pp.243-288.
Kabaciński, J. and Winiarska-Kabacińska, M., 2023. An inverted perspective: Identification of stone tools used in the production of bone and antler artefacts in the North European Mesolithic-the case of the Dąbki site, Poland. Quaternary International, 668, pp.27-43.
Kufel-Diakowska, B., 2011. The Hamburgian Zinkenperforators and burins-flint tools as evidence of antler working. In: J. Baron and B. Kufel-Diakowska, eds. 2011. Written in Bones. Studies on Technological and Social Context of Past Faunal Skeletal Remains. Wrocławski: Uniwersytet Wrocławski, Instytut Archeologii. pp.233-242.
Langley, A. and Wisher, I., 2019. Have you got the tine? Prehistoric Methods in Antler Working. EXARC Journal, [online] Available at: < https://exarc.net/issue-2019-2/at/have-you-got-tine > [Accessed 13 September 2022].
MacGregor, A., 1985. Bone, antler, ivory & horn: the technology of skeletal materials since the Roman period. London: Croom Helm.
Maté-González, M., Yravedra, J., González-Aguilera, D., Palomeque-González, J., and Domínguez-Rodrigo, M., 2015. Micro-photogrammetric characterization of cut marks on bones. Journal of Archaeological Science, 62, pp.128-142.
Maté-González, M., Palomeque-González, J., Yravedra, J., González-Aguilera, D. and Domínguez-Rodrigo, M., 2016. Microphotogrammetric and morphometric differentiation of cut marks on bones using metal knives, quartzite, and flint flakes. Archaeological and Anthropological Sciences, 10(4), pp.805-816.
Maté‐González, M., Yravedra, J., Martín‐Perea, D., Palomeque‐González, J., San‐Juan‐Blazquez, M., Estaca‐Gómez, V. and Domínguez‐Rodrigo, M., 2018. Flint and quartzite: distinguishing raw material through bone cut marks. Archaeometry, 60(3), pp.437-452.
Molloy, B., Wiśniewski, M., Lynam, F., O'Neill, B., O'Sullivan, A. and Peatfield, A., 2016. Tracing edges: A consideration of the applications of 3D modelling for metalwork wear analysis on Bronze Age bladed artefacts. Journal of archaeological science, 76, pp.79-87.
Orłowska, J., 2015. How Did They Drill That?-A Few Observations on the Possible Methods for Making Large-sized Holes in Antler. EXARC Journal [online] Available at: < https://exarc.net/ark:/88735/10193 > [Accessed 13 September 2022].
Pratsch, S., 2011. Mesolithic antler artefacts in the North European Plain. In: Written in Bones. In: J. Baron and B. Kufel-Diakowska, eds. 2011. Written in Bones. Studies on Technological and Social Context of Past Faunal Skeletal Remains. Wrocławski: Uniwersytet Wrocławski, Instytut Archeologii. pp.79-92.
Riedel, K., Pohlmeyer, K. and Von Rautenfeld, D., 2004. An examination of Stone Age/Bronze Age adzes and axes of red deer (Cervus elaphus L.) antler from the Leine Valley, near Hannover. European Journal of Wildlife Research, 50(4), pp.197-206.
Sapirstein, P., 2018. A high-precision photogrammetric recording system for small artifacts. Journal of Cultural Heritage, 31, pp.33-45.
Smith, C., 1989. British Antler Mattocks. In: C. Bonsall, ed. 1989. The Mesolithic in Europe: Papers Presented at the Third International Symposium. Edinburgh: John Donald Publishers Ltd. pp.272-283.
Tolan-Smith, C. and Bonsall, C., 1999. Stone Age studies in the British Isles: the impact of accelerator dating. Mémoires de la Société préhistorique française, 26, pp.249-257.
Van Gijn, A., 2014. Science and interpretation in microwear studies. Journal of Archaeological Science, 48, pp.166-169.
Zupancich, A., Mutri, G., Caricola, I., Carra, M., Radini, A. and Cristiani, E., 2019. The application of 3D modeling and spatial analysis in the study of groundstones used in wild plants processing. Archaeological and Anthropological Sciences, 11(9), pp.4801-4827.
Zvelebil, M., 1994. Plant use in the Mesolithic and its role in the transition to farming. Proceedings of the prehistoric society, 60(1), pp.35-74.