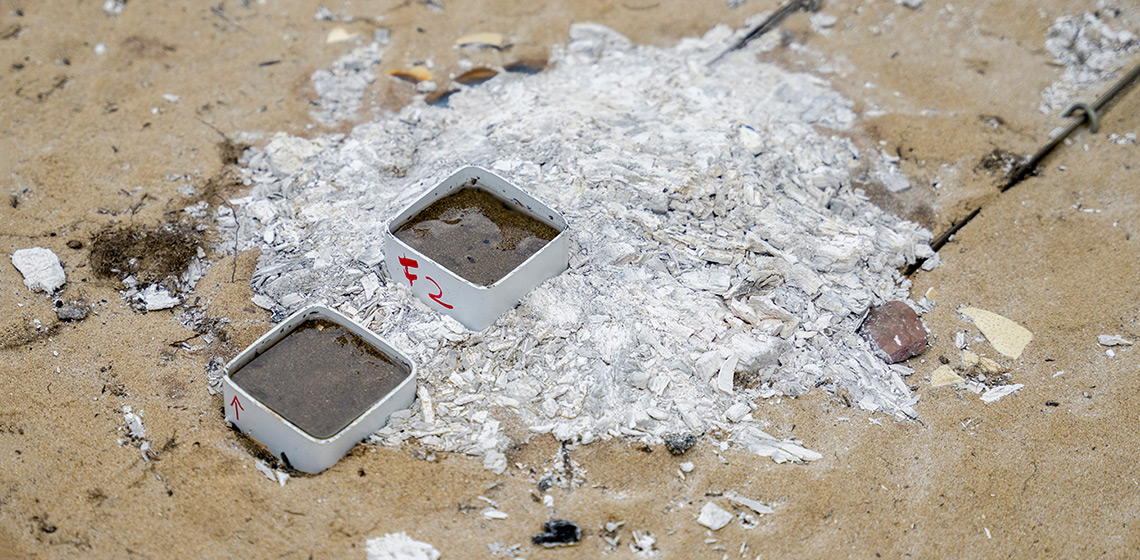
Hearth formation processes are complex. They involve multiple actions, reactions, and activities before, during, and after an active fire and can also impact a wide range of materials and sediments at an archaeological site. Archaeologists approach combustion features and formation processes from multiple analytical perspectives. However, many experimental studies are limited to a strict analytical focus on a single or very few fire-related aspects to allow for careful control of specific variables. Six researchers report here on a multi-focus experimental approach in order to understand complex fire practices and heat-induced alteration of micromammals, ostrich eggshell fragments, mineral pigments, shellfish, and sediments. We designed and conducted five experiments with a state-of-the-art 3D documentation setup, active fires, and excavation through photogrammetry and spatial recording. We provide a brief general account of the experiments and an overview of the experimental design before comparing single-focus and multifaceted experiments and pointing to some of the benefits of our approach and potential areas for improvement. Multifaceted experiments are complex and resource-demanding, and proto-experiments should ideally be part of the experimental design. Our 3D recording and collaboration on documentation strategies provided a wide range of data that can further our understanding of prehistoric combustion features.
Introduction
Examining the distribution of burnt remains and sediments is important for evaluating the location, preservation, and function of hearths and combustion features in the archaeological record (Berna and Goldberg, 2007). Archaeologists, therefore, regularly use the presence of ash and charcoal, as well as the size, placement, and frequency of hearths, to infer prehistoric fire-related human behaviour (e.g., Shahack-Gross et al., 2014; Vallverdú et al., 2012; Wadley, 2006). At a hunter-gatherer campsite, a fireplace represents one of few structural elements around which the rest of the campsite is organized. As a natural focal point for campsite activities – including socialization, toolmaking, and cooking – both the placement, content, and structure of a fire can therefore reveal much information on the function of the fire, its makers, and their activities around the campfire.
Hearth formation processes, as an archaeological term, stricto sensu refers to all processes occurring during or shortly after the stage of active combustion in a fireplace (see also definitions and discussions in Aldeias et al., 2016; Bentsen, 2014; Berna and Goldberg, 2007; Mentzer, 2014). Most of the intentional effects of the fire happen during this stage; many of these effects tend to be both momentary and abstract in nature. Examples of intentional effects include warmth, light, smoke, fire as a tool in heat treatment or cooking, or any combination of these (e.g., Bentsen, 2014). Additionally, a campfire may also cause a range of unintentional effects, such as the gradual and physical accumulation of ash and charcoal or the heat-induced and often non-reversible alteration of the substrate below a fire. In archaeological contexts, linking the abstract intentions or functions of a fire with the physical remains of combustion can be quite challenging as fire-related processes can manifest themselves in both visually detectable ways and subtle, visually unrecognizable ways.
Hearth formation processes thus cannot be regarded as a simple process or as a single activity. If a fire is used more than once or over a long period of time, for example, the hearth formation processes can become exceedingly complex as multiple generations of combustion are stacked on top of each other, resulting in a partial but continuous reworking of the hearth feature. Subsequent cleaning of a burnt-out fire, for example, by raking out the ashes, can also alter the content and shape of the original hearth structure (Mentzer, 2014). Consequently, the planning, burning, and maintenance of an anthropogenic fire constitute a multi-dimensional stack of events that are dynamically shaped through an idiosyncratic mix of practical and cultural choices, local and environmental variables, complete and incomplete combustion and are subject to a range of post-depositional processes. While the configuration of hearth features in archaeological contexts may appear simple in the field – most often characterized by a layer of charcoal below a layer of ash – the variables involved in their making are the product of multifaceted human behaviour combined with variable thermal and geochemical reactions (Mallol et al., 2007; McCauley et al., 2020). To robustly recognise and decipher the key variables involved in prehistoric hearth formation can therefore be exceedingly challenging.
Archaeologists have approached the complex nature of prehistoric combustion features from multiple analytical perspectives and have adopted physical and behavioural hearth formation models from a range of fields, including ethnography, geoarchaeology, palaeobotany, faunal taphonomy, material science, and social theory. Particularly within Palaeolithic research, there has, during the last decade, been an increased focus on the use of pre-arranged actualistic fire experiments in order to better understand how different hearth features are formed and to assess which variables govern the process (Aldeias et al., 2016; Aldeias et al., 2019; Bentsen, 2012; 2013; Hoare, 2020; Mallol et al., 2013a; Mallol et al., 2013b; Miller and Sievers, 2012; Sievers and Wadley, 2008). While these experiments have provided a wealth of new and important information, most of them have been designed and executed by few participants and within a limited analytical framework to allow for stricter control of specific variables. The focus of most actualistic experiments has, consequently, been on a single activity or a carefully chosen process. The limited range of activities represented in each study, unfortunately, excludes the possibility of conducting more comprehensive analyses of the combined effect of simultaneous fire-related actions. Furthermore, inter-experimental comparison between analytically narrow and highly specialized fire experiments may not always be possible due to variations in documentation and sampling methods.
In his comprehensive study of experimental fires, Karkanas (2021) argues that the only way we can fully understand and correctly interpret archaeological campfires is by adopting interdisciplinary research strategies capable of assessing the analytical limitations, potentials, and vulnerabilities of different types of fire-related proxies. Furthermore, Karkanas (2021) emphasizes that more experimental fire studies are needed, particularly those that focus on improving our understanding of the combined behavioural, mechanical, thermal, chemical, mineralogical, structural, and morphological aspects of hearth formation processes. Our paper presents an experimental study within the growing corpus of interdisciplinary experimental archaeology. This research field recognises that the complex nature of both prehistoric and experimental remains ought to be documented, analysed, and interpreted through a range of perspectives, methods, and concepts. When this type of multi-focus experimental approach is implemented into the design of an archaeological experiment from the beginning, it allows the participating researchers to document, analyse, and interpret a much broader range of proxies and observational data than in standard single-focus experiments. Multifaceted experiments consequently increase our chance of robustly inferring complex fire practices through burnt remains.
For the experimental study (referred to as the pilot season) described here, we relied on the combined knowledge of six researchers. Each researcher had their own areas of expertise and background (See Table 1) that were used to create a combined interdisciplinary experimental methodology. The setup of the fires was carefully designed to promote transparency and reproducibility, and each fire was monitored and documented using state-of-the-art documentation and excavation techniques. The team members were experts on different materials and methods – e.g., prehistoric pigment use, shellfish collection, faunal taphonomy, micromorphology, experimental archaeology, and spatial recording methods – with an interest in conducting actualistic fire experiments for different (disciplinary) reasons (See Figure 1 and schematic overview in Table 1). Preliminary results from most of the subprojects were presented at the EAC12 World Tour conference (Bentsen, 2021; Haaland, 2021; Nel, 2021; Unhammer and Armitage, 2021), and we focus here on more general and methodological observations.
Name | Background | Research interests here |
Silje Evjenth Bentsen | Experimental archaeology | Heat-induced changes (OES, sediments) |
Pyrotechnology | Fire behaviour | |
Project management | Methodology | |
Magnus Haaland | Site formation processes | Heat-induced changes (long-term fires) |
Micromorphology | Sampling strategies, preparation and collection | |
Spatial analysis | Microcontextual analysis | |
Jovana Milic | Stable isotopes | Heat-induced changes (shellfish) |
Paleoclimate | Stable isotopes | |
Shellfish analysis | Experimental archaeology | |
Turid Hillestad Nel | Micromammals | Heat-induced changes (micromammals) |
Palaeoenvironment | Fire-related formation processes | |
Avian predators in archaeological contexts | ZooMS | |
Ole F. Unhammer | Photogrammetry | Photogrammetry |
Spatial recording and analysis | Methodology | |
Digital recording | Experimental archaeology | |
Elizabeth Velliky | Prehistoric pigment use | Heat-induced changes (mineral pigments) |
Geochemistry | Fire-related formation processes | |
Provenance studies | Methodology |
Table 1. Overview of the experimental team, including background and research interests.
In this paper, we showcase how each perspective and disciplinary know-how contributed to shaping the overall design of our experiments. We provide a brief general account of the pilot season, including the various stages of preparation, execution, and documentation, as well as an overview of experimental designs and examples showing the practical and analytical potential of interdisciplinary experimental data integration. Towards the end of the paper, we compare multifaceted and single-focus experiments through examples from our pilot season, point to the benefits of our approach, and potential areas for further improvement.
The experimental field season
The pilot season included a preparation and planning phase of 8 months, an execution and documentation phase of 3-4 weeks, including practical setup and cleaning, and a still ongoing analytical phase. It is vital to recollect this temporal perspective as we provide a general outline of the stages of the pilot season below while illustrating examples through our Experiment 4 (E4).
Preparation – experimental design
The initial planning and preparation of the experiments included several team meetings to ensure that the overall project design was informed by a range of perspectives and compatible with multiple research agendas. Each team member had their own subproject (See Table 2) with specific research aims and strategies for sampling and documentation, which were described in detail in a separate sub-project plan. We conducted several joint reviews of these plans to ensure that the different experimental concepts were robustly aligned with each other, analytically, logistically, and in terms of documentation requirements. During this iterative quality-check process, significant milestones and mutual recording procedures were identified and formulated within the overall project plan. We developed standardized documentation forms to ensure consistent recording and documentation during all experiments. In essence, our collaborative planning and review process facilitated continuous refinement of both overall and subproject-specific research design (including the individual analytical steps taken), which in turn led to an overall improvement of the entire pilot season.
Experiment | Main aim | Duration manual logs (hours) | Material in sediments | Material on surface | No of thermocouples per fire |
E1 | Test equipment and concept | 12 | No | No | 6 |
E2 | Hearth management | 253 | Yes, at -5 cm | Yes | 2 |
E3 | Shellfish cooking | 8 | Yes, at -5 and -10 cm | Yes | 4 |
E4 | Owl pellets containing micromammals in the fire and as tinder | 20 | Yes, at -5 and -10 cm | Yes | 4 |
E5 | Heating effects OES | 102 | Yes, at -5 and -10 cm | Yes | 4 |
Table 2. Overview of the five experiments, including main aim for each experiment.
The complete plan for the entire experimental project consisted of five separate and consecutive experiments (See Table 2), where the focus of each was initially motivated by the disciplinary agenda of one team member. Nevertheless, the planning phase modified and streamlined the experiments to include analytical elements from other sub-projects. For example, while experiment number 4 (E4) focused on the ignition capabilities and heating response of owl pellets containing micromammals, other types of material such as shellfish, ostrich eggshell fragments (OES), and ochre were incorporated so that we could also evaluate their thermal alterations.
The control of variables is an essential part of scientific experiments (e.g., Outram, 2008). An important part of the experimental planning was, therefore, to review and include measures to enhance experimental control. Parallel fires conducted simultaneously during an experiment have, for example, proven a productive approach to ensure similar conditions and control for unexpected events (Bentsen, 2012; 2013; Tietema et al., 1991). We, therefore, decided that each of the five experiments was to include two simultaneous fires (F1, F2) with an identical setup in terms of a substrate as well as data preparation and documentation, thus making sure that we could maximise research output. To cite an example, the two fires in E4 had an identical basic setup and were active simultaneously, with similar conditions and the same fuel mass. However, only one of the two fires (F1) of E4 included owl pellets on the surface to simulate accumulation after roosting activities and/or site maintenance.
To achieve robust spatial control over sediments, material, and physical properties such as heat distribution and moisture content, we designed and created a standardized physical recording environment – a fixed-sized sandbox – in which the experiments would be conducted (See Figure 2). The known format of the boxes allowed us to pre-plan each individual experiment in terms of their spatial layout and material integration. The design of, and results from, previous experiments (e.g., Bentsen, 2012; 2013; 2014; Mallol et al., 2013b; Sievers and Wadley, 2008) have demonstrated that a 100 x 100 cm surface area would be sufficient for our experiments and a substrate thickness of 30 cm would accommodate for the predicted alterations occurring below the fire. Our boxes thus had an interior base of 100 x 100 cm and a height of 30 cm. The modular design allowed for easy packing, transportation, and assembly and ensured that the experiments could be conducted in any location with minimal impact. Four identical boxes were constructed and used during the experiments and can be reused in the future.
Preparation - logistics
The pilot season was to be conducted in South Africa, while all the team members were based in Europe (Norway). Long-distance planning was, therefore, necessary, which created a few challenges. For example, locating a suitable venue for the experiments and acquiring all the different tools, recording devices, and materials needed had to be pre-arranged from a distance. For this reason, a network of local contacts had to be established, and they proved instrumental in providing administrative and logistical support. Local collaboration for E4 included close cooperation with the U3A Bird Group in Still Bay and its leader Johan van Rooyen who, in cooperation with local farmers, identified various barn owl (Tyto alba) locations in the Hessequa area and collected owl pellets from these sites. The pellets consist of bone and fur from the owl’s prey, where rodents such as mice, gerbils and shrews are abundant.
After some deliberation, it was decided that the pilot season was to be conducted in Jongensfontein Caravan Park, located along the southern Cape coast in Jongensfontein, South Africa, some 15 km southwest of Still Bay (See Figure 3). We were not able to visit the park before conducting the pilot season there. Without being familiar with the facilities, it was difficult to predict the amount of space available for hosting the fire experiments, as well as how the other campsite residents would react to having five relatively continuous fires creating smoke for three weeks.
Three cabins were rented, with a small space in between to conduct the fires (See Figure 4). The cabins were relatively offset from the rest of the park, which was beneficial to avoid disturbing the other caravan park residents with the high smoke load. No complaints were received from the other residents, although we were told that some moved their caravans to avoid the smoke from the experiments. We did, however, receive several visits from curious neighbours inquiring about the experiments and the archaeology of the area. Consequently, having the experimental season in a well-visited caravan park proved to be a great public outreach opportunity. In addition to a physical presence and outreach at the caravan park, we also ran a digital outreach component, including a blog with text, photos, and movie clips (Bentsen 2020) as well as updates on social media. The digital outreach programme was well received and made it possible for anyone anywhere to follow our progress.
Execution
We arrived in South Africa in February 2020 and spent nearly five days buying, collecting, testing, and packing equipment before leaving for Jongensfontein, where we arrived on the 16th February. The pilot season, including the time spent on setup and testing in Jongensfontein, the active experiments, and the subsequent cleaning and packing up, lasted for 21 days.
We started the pilot season by assembling the boxes and mapping their locations with a total station (See Figures 5 and 6). After the initial setup, the coded ground control points were put directly on each side of the boxes and made the photogrammetric record of the box and its content more streamlined (See Figure 7). The boxes were filled with two types of sand. We purchased in total 1 m3 of industrial sand for use as the base layer and collected nearby dune sand as the top 15 cm layer for each box (See Figures 8 and 9). The quantity of industrial sand was sufficient for the entire season as we did not have to remove and replenish large quantities of the base layer between each experiment. The heat-altered dune sand covering the top 15 cm of the boxes, however, was removed after each experiment as part of excavation and documentation procedures and to ensure identical sedimentary and substrate conditions for the next experiment.
Four of the experiments carried out during the pilot season included shellfish, OES, mineral pigment material (ochre), micromammal bone fragments, and/or owl pellets. We distributed this material on the surface of the fire and also buried material at -10 cm and -5 cm below the surface (See Figure 10, Table 3). The subsurface depths are comparable to those used in other experiments (e.g., Sievers and Wadley, 2008). The 3D position of deposited materials was recorded with a total station upon deposition, before the fire, and upon recovery during the post-fire excavation.
Items at -15 cm | Items at -10 cm | Items at -5 cm | Items at surface | ||||||||||||||||||||
Experiment | Fire | Wood type | Total wood weight (kg) | Thermocouple | OES | Micromammals/pellets | Shellfish | Mineral pigments | Thermocouple | OES | Micromammals/pellets | Shellfish | Mineral pigments | Thermocouple | OES | Micromammals/pellets | Shellfish | Mineral pigments | Thermocouple | OES | Micromammals/pellets | Shellfish | Mineral pigments |
1 | 1 | Acacia cyclops | 3 | 1 | 1 | 1 | 3 | ||||||||||||||||
2 | Vachellia erioloba | 3 | 1 | 1 | 1 | 3 | |||||||||||||||||
2 | 1 | Acacia cyclops | 730,1 | 0 | 0 | 1 | Y | Y | Y | 1 | Y | ||||||||||||
2 | Acacia cyclops | 687,8 | 0 | 0 | 1 | Y | Y | Y | 1 | Y | |||||||||||||
3 | 1 | Vachellia erioloba | 6 | 0 | 1 | Y | Y | 1 | Y | Y | 2 | Y | |||||||||||
2 | Vachellia erioloba | 6 | 0 | 1 | Y | Y | 1 | Y | Y | 2 | Y | ||||||||||||
4 | 1 | Vachellia erioloba | 6 | 0 | 1 | Y | Y | Y | Y | 1 | Y | Y | Y | Y | 2 | Y | Y | ||||||
2 | Vachellia erioloba | 6 | 0 | 1 | Y | Y | Y | Y | 1 | Y | Y | Y | Y | 2 | Y | Y | |||||||
5 | 1 | Vachellia erioloba | 187,5 | 0 | 1 | Y | Y | Y | 1 | Y | Y | Y | 2 | Y | Y | Y | |||||||
2 | Vachellia erioloba | 188,8 | 0 | 1 | Y | Y | Y | 1 | Y | Y | Y | 2 | Y | Y | Y |
Table 3. Detailed overview of the setup of all experiments.
The temperatures of the fires were recorded using two EXTECH SDL200 4-channel thermometers (experiments 3, 4, and 5), two Huato S220-T2 Dual-channel thermometers (experiment 2), or all four thermometers (experiment 1). Both thermometer models are digital data loggers and were set to capture temperatures at 30-second intervals. The thermometers were connected to K-type thermocouples, which were custom-made for us by DLD Scientific. The number of thermocouples and depths for temperature recording for each experiment are summarised in Table 3 and illustrated in Figure 11. The position of each thermocouple was recorded by the total station before each fire and during excavation. In addition to the digital temperature recording, we conducted manual temperature recordings of the fires every hour until the temperatures were declining and approaching 100ºC or below. The main advantage of digital data loggers is the automatic recording and precision of the temperatures. However, a thermocouple will only register the temperature at its tip in an area that can be < 1 mm in diameter. The thermocouple and data capture are thus sensitive to movement, sediment changes or sudden weather fluctuations such as strong wind. While conducting manual temperature recordings, we also controlled the thermocouples and recorded the fire with surface photos. Furthermore, we recorded the weather conditions in terms of air velocity, humidity, and air temperature hourly using an EXTECH 45158 handheld anemometer. These data provide essential control points for the analysis of temperature developments during the fires.
Two woody taxa were used for the experimental fires (See Table 3). Vachellia erioloba, previously referred to as Acacia erioloba, commonly named Kameeldoring in Afrikaans or Camel Thorn in English, is indigenous to southern Africa, occurring inland on sandy loams up to central Africa (Palgrave, 1977). This wood was selected as it is indigenous, known to generate much heat, and available in suitable quantities in proximity to our base. The taxon has been used in previous fire experiments or studies of firewood properties (e.g., Bentsen, 2014; Munalula and Meincken, 2009; Schmidt et al., 2015; Wadley and Prinsloo, 2014), allowing for comparisons. The second taxon used, Acacia cyclops, commonly named Rooikrans in Afrikaans or Coastal wattle in English, is an alien invasive shrub from Australia, now widespread in South Africa (Post et al., 2010). Rooikrans was selected due to its vast availability as we required a large quantity of wood for the duration of experiment 2 (See Table 3). Previous studies have examined the properties of Rooikrans as firewood (e.g., Meincken, 2009; Munalula and Meincken, 2009), and the taxon is considered an excellent fuel (Smit and Meincken, 2012). We recorded the moisture contents of a sample of the wood used in the experiments with an EXTECH hygrometer. The average wood humidity for Vachellia erioloba was 9.2%, and the average for Acacia cyclops was 15.5%.
The fires needed careful monitoring in addition to the detailed recording and documentation performed once an hour. Consequently, the project manager made a detailed duty roster to organise responsibilities, including the recording of the prevailing weather conditions, refuelling of the fires, and the visual documentation through photography. See Figure 12 for an overview of the documentation procedures conducted every hour during the active experiments. Each experiment was led by one person, who coordinated the setup and start of the fires, including pre-planned tasks such as putting shellfish or ostrich eggshells in the fire or sweeping ashes, while other members assisted in recording these events. Because two experiments ran in parallel for most of the season, up to four active fires (two for each experiment) were maintained, documented and/or excavated at the same time (See Figure 13).
Post-burning sampling and excavation
Once the fires had stopped, and the surface of the ashes had cooled down sufficiently, the process of digital and spatial documentation, sampling, and excavation could commence. Both before and during subsequent stages of excavation, the burnt-out hearth surface was recorded in 3D using digital photogrammetry (See Figure 7). The experiment (box) surface was divided equally into two 50 x100 cm rectangles, and a set of 2-4 micromorphological sampling tubes were vertically inserted into the hearth centre within the southern rectangle (See Figure 14). The two rectangles were mechanically separated using a transparent glass plate that helped to stabilise the loose sediments and preserve the profile of the remaining half.
The stratigraphic excavation of the experimental hearth features was documented with conventional overview photos, photogrammetric 3D surface recordings, and detailed excavation notes (See Figure 15). A Munsell Colour Chart was used to identify and describe the appearance of the excavated sediments. Our general excavation procedures were as follows. We first removed the loose ash and charcoal from the top of the hearth, measured its volume, and sampled it. If there were samples of OES, mineral pigments, and/or owl pellets on the surface, these were documented by photogrammetry before removal. After exposing the ash crust beneath the loose ash, we documented and sampled it and excavated it to the ash base. The layers beneath the ash base were then excavated stratigraphically, following the natural (basin) shape of the hearth. To preserve the structural integrity of the hearth during excavation, moisture was carefully introduced to the sediments. If a particular hearth layer was thicker (deeper) than 5 cm, its photogrammetric recordings were taken at every 5 cm during excavation. We collected loose sediment samples for every type/colour of sediment and recorded coordinates of the positions from which we collected the samples.
Once the first half of the hearth was fully excavated, its profile was recorded using photogrammetry through the transparent dividing plate (See Figure 14B and D). Moisture was then carefully introduced into the sediments of the second half to improve stabilisation. The excavation of the second half of the hearth followed the same protocol, with a special focus on recovering the material placed in the sediments at 5 cm and 10 cm below the surface (See Figure 14F). This meant careful excavation, ensuring that all micromammal bones were recovered, and the sediments were sieved (1 mm mesh-size) during removal.
Analytical potential
The data collection and documentation strategies were chosen to ensure analytical possibilities for our individual material, samples, and experiments. The integration of interdisciplinary experimental data enabled a broader, multifaceted perspective for analyses of results. To illustrate the potential of integration of multi-dimensional experimental fire data, we will use E4 Fire 2 as an example. This fire had owl pellets containing micromammals placed on the surface of the dune sand (See Figure 16) and was otherwise set up as described above (See also Figure 10 and Table 3), with shellfish, OES, owl pellets, micromammals, and mineral pigments at -10 and -5 cm, and OES and mineral pigments on the surface.
The photogrammetric recording was carried out before and during the active part of the fire experiment, as well as at regular intervals during the post-fire excavation (See Figure 7). While we are still in the progress of synthesizing this data, it is already clear that this method provides a wealth of information. Especially in terms of documenting precisely the placement and potential movement of the material embedded below and in the fire, as well as the spatial relation between the temperature probes and the location of combusted material.
Photogrammetry also served as a great method for recording the general development of the fire over time. Its capability to produce spatially accurate and visually intuitive results of the fire, above and below the ground, makes it a powerful exploratory and analytical tool that is excellently suited to documenting complex archaeological experiments. The method enables illustration of the experiment from start to end, providing valuable visual, spatial, and contextual data that, when combined, make it easier to interpret results (See Figure 17). The resulting visual models of different stages of the fire can be used, for example, to examine the thermal and physical development of the fire, how the flame position migrates over time, and how visual changes in OES and pigments on the surface appear and evolve.
The various materials placed in the sediments, which are individually informative, can in cohesion be used for correlation of observed variations and thermal responses, not just within the same fire experiment but also across the different experiments. The materials form reference collections on a micro-scale within one experiment and sediment depth and on a macro-scale for the entire assemblage of OES, micromammal fauna, ochre, and shellfish from the experiments.
The micromorphological samples collected from the fire experiments provide information on the sedimentary content and internal microstructure of the fires, as well as the effects of heat on the substrate. Oriented block samples provide a unique, in situ glimpse of the sedimentary signatures associated with various hearth- and fire-related activities (See Figure 18).
Discussion
The general milestones and overall project design of multifaceted experiments, as described above, and of standard single-focus experiments are similar. Both experiment types include phases of planning, execution, and analysis and involve the development of schedules and deadlines for buying equipment and the expected start and end of the project. There are, however, both subtle and distinctive differences between the experiments, and we will discuss some examples and compare the experiment types below. In doing so, we will also point to the benefits of our approach and potential areas for further improvement.
General observations
The level of complexity differs between the two experiment types. A single-focus experiment, on the one hand, normally involves only selected processes and activities. From this follows that the standard single-focus experiment incorporates few critical moments or milestones that must be performed at a certain time or within a specific context. This type of experiment may thus allow for some flexibility in risk management as it is easy to get an overview of issues as they appear. The ripple effect of any delays would be limited, as a limited number of processes are involved, and one might be able to counter potential negative effects entirely through pre-experiment risk assessments and swift action during the experiment. Multifaceted experiments incorporating several disciplines and researchers, on the other hand, include added critical milestones and operational sequences that need to be performed in a set order. The setup of E4 for the pilot season, for example, involved coordinating and documenting the placement of OES, shellfish, ochre, and microfauna at specified depths. Delays in adding one of these elements would affect the next step in the process and could start a ripple effect that affected several processes from the start of the fire to the management of the fire and the excavation of the hearth, and that could also affect the schedule for the following experiment.
The existence of many critical moments in an experiment can be challenging. However, the added complexity is counterbalanced by an added collective experience better equipped to predict potential challenges or critical moments and to avoid and correct any issues. During the pilot season described here, we could draw on the knowledge of different researchers to use and correct any problems with thermometers, cameras, and other types of equipment within a reasonable timeframe because the team included people with intimate knowledge of our tools (See Figure 19).
Both multifaceted and single-focus experiments produce high-quality data. Nevertheless, there are potential differences in the data corpus generated by these experiment types. The single-focus experiment typically produces large quantities of data on a singular aspect of the fire. The multifaceted experiment, in contrast, aims to generate data on multiple aspects and thus facilitates comparisons of different types of materials or phenomena under study. One of the main challenges for all types of multifaceted experimental projects, however, is how to efficiently integrate diverse datasets that are inherently different in their nature (e.g., spatial, visual, three-dimensional, behavioural, macroscopic, microscopic), that has been recorded over different time depths (before, during, after active combustion), at different locations (different surface levels in the field, observations conducted in a laboratory), and using a range of different analytical techniques (e.g. field descriptions, photos, plotted finds, 3D topography, micromorphology, FTIR, temperature data, environmental data etc.). Experimental data integration from multi-focus experimental projects often boils down to the very practical questions of how to link different types of field data and how to combine results more intuitively during the exploratory and analytical phases after the experiments have been conducted. The documentation solutions and experimental designs for the pilot season proved to promote transparency and reproducibility as well as interactive, visual, and intuitive data sharing and exploration amongst all participants.
Preparation and planning
The preparation and planning phase of all scientific experiments should contain methodological reviews and the development of innovative, appropriate, and productive perspectives to explore the selected research questions. Informal or formal colloquies, feedback meetings, or other discussions with collaborators and fellow scientists are essential parts for maturing the experimental strategies. Nevertheless, this process is more extensive for multifaceted experiments with more aspects to review, thus potentially also more feedback and synergistic effects in refining methods and parallel processes. As described above, for example, our planning phase included several meetings where we discussed operational sequences that needed to be streamlined. We made plans to ensure that the photogrammetric documentation captured all important aspects of the fires yet avoided redundant repeat documentation of minute details.
A potential pitfall in the streamlining of operational sequences and methods is, furthermore, that minor yet essential, procedures for one part of the project can be removed or distorted during the review process. Our frequent meetings, detailed plans for subprojects, and review of the general experimental design ensured that all vital stages were included in the final plans. The planning process was time-consuming and resource-demanding but ultimately essential to augment the scientific integrity of the pilot season.
We described certain logistical challenges above. The challenges were on multiple levels, from not having sufficient local knowledge to establish where wood for the fires could be sourced to finding a suitable location for accommodating our experiments. In instances where we could not rely on an established local network of people, we had to accept that problems needed to be solved upon arrival or ad hoc during our project. The latter required the flexibility and ingenuity of the project plans and the researchers involved. Accommodating time and planning for these unforeseen events were naturally challenging, also when considering that extending the project length was not an option. For the project leader and as a group, this required a stern focus on the planned output of our experiments while adjusting these goals and planned activities when unforeseen delays occurred. Pre-planning extra time to organize and address emerging issues on location was key for the success of our project.
In evaluating the pilot season, we find that added proto-experiments or detailed pre-training of the experimental team would have been beneficial for several reasons. Firstly, it would provide team members with a better intimate understanding of the experimental procedures and sequences and the critical moments in each experiment. Secondly, it would provide all team members with an increased awareness of the parameters and variables applied in and essential to the experiments, as well as the recording thereof. Since we had fires that at times needed to be maintained and supervised by one person alone, for example, each member needed to know how to use and read different measuring devices such as hygrometer, anemometer, thermometer, and total station, and to perform the photogrammetric imaging capture workflow. Thirdly, and the last example here, is that it would equip team members with the practical skills required for the thorough redesigning or redeveloping of their experiment. For the pilot season, the training phase coincided with the beginning of our first two fire experiments. However, this sort of training can be planned and carried out well in advance before the commencement of archaeological experiments. The need for pre-training is especially seen in the most complex operations. For example, most of the photogrammetric recording was conducted successfully, but there were a few cases where insufficient or inadequate images had been collected. This was caused by an insufficient understanding of the method, procedures, or equipment and could have been prevented with more training. Training should preferably include multiple theoretical and practical sessions, allowing for the necessary practice to develop the essential understanding, routines, and confidence needed for autonomous recording.
Execution of experiments
The project design and sheer manpower available allowed complex long-term experiments that contributed to the understanding of how different activities and operational sequences are entangled during complex hearth formation processes. The dynamic of shift arrangements enabled each researcher to focus on their experiment while also gaining in-depth knowledge of the other experiments taking place. The active role of each researcher was particularly important in securing communal knowledge and ownership to all components of the project while also facilitating the transfer of skills. However, the supervision and maintenance of such experiments can be stressful and include monotonous tasks that require a high level of attentiveness and diligence. The shift arrangements included both day and night duties, which meant that the team members could not follow their normal routines. All team members did get some time to unwind, and we did have extra hands to help with shellfish collection and kitchen duties. Nevertheless, the workload and disturbed sleep patterns could potentially contribute to distress and other mental reactions. Although there are great benefits with complex, multifaceted experiments, they are highly labour and resource-intensive, particularly the long-duration fires. Careful consideration of research output vs. labour and resource requirements are advised for future projects of similar design, and a detailed schedule is essential to the workflow.
Experimental archaeology, at its core, provides great opportunities for interaction with the general public and the transfer of practical skills internally between members of the group conducting the experiments as well as external skill transfer between the team members and others. Our pilot season, in a public caravan park, allowed us to interact with others, explain what we were doing, and get feedback from the public on practical issues such as wood taxon properties or cooking procedures. In addition to the in-person interactions on-site, we also updated our online presence (Bentsen, 2020). While the physical outreach could take place as we were performing activities and within the natural flow of the experiments, the digital outreach required sitting down to find photos, make videos, write and edit texts, and taking the time to deal with any technical problems such as issues with the login for our blog platform or internet connectivity. The digital outreach proved to be the most time-consuming and labour-intensive, even if there was a division of tasks between participants. The digital outreach could, nevertheless, have been organised better to save resources, for example, by the delayed posting of the season or adding an extra person that could focus on the outreach part of the pilot season.
The experimental boxes proved one of the most successful components of the pilot season. They were very easily assembled and provided stable environments for the fires. Even so, it was nearly impossible to move the boxes once filled with sand. The placement of boxes and fires thus had to be carefully considered at the start of the season. Furthermore, we made labels for the boxes to distinguish each fire and for photogrammetric purposes, and some of these labels had to be replaced during the season. It was not a time-consuming process but provided a new set of critical moments that must be included in future plans.
Analysis of experiments
The photogrammetric recording provides an advantage over conventional drawing, measurement, and photography in that it can collect all this information into a single 3D model. The photogrammetric recording thus provides a highly precise, detailed, and flexible dataset that encompasses information necessary for the study of all the different individual experiments and a precise spatial record in which we can confidently study the spatial relations between material, measurements, and the active fire. Finalising the models requires, however, both suitable software and the skills to use the software. When such resources are available, the photogrammetric model provides an extra level of data and is a highly productive addition to other recording methods.
The pilot season involved individuals documenting data for each subproject, such as details on the microfauna in each fire, as well as the communal collection of data and metadata from each fire, such as weather conditions or the number of photos. Digital data, for example, photos or logs from the thermometers, needed to be organised or entered into databases so that the context of the data was preserved. Manual weather recordings or notes needed to be digitised. Both the digitised and digital data had to be uploaded to repositories and made available to all team members before further analyses could be completed. Due to the pandemic, we had time to complete the digital maintenance of data within a short time after the completion of the pilot season. Data thus became available to all researchers, which was vital to the progress of all analyses. This underlines the need to allocate time for data processing and digitising post-experiment.
Conclusion
In this paper, we have demonstrated how an interdisciplinary experimental approach to actualistic fire experiments can strengthen both the design and the analytical power of the experiments by promoting a more comprehensive integration of observational data in time and space. The complexity of hearth formation processes was replicated by complex, multifaceted experiments. The diversity of the SapienCE experimental team allowed for the planning and execution of interdisciplinary fire experiments on a larger scale and with more comprehensive documentation than what is currently the convention for these types of studies. The setup of the fires was carefully designed to promote transparency and reproducibility, and each fire was monitored and recorded using state-of-the-art documentation and excavation techniques. Lastly, we also want to promote transparency between research teams in publishing here a detailed record of our experimental approach and evaluations of our season.
Acknowledgements
We are grateful for all the support received during all phases of the pilot season and only have space to mention here those that provided extra support. Special thanks to the U3A Bird Group in Still Bay and its leader Johan van Rooyen for the help in collecting owl pellets and the landowners Annamarie Barnard, Kobus Groenewald, Dirk Hopkins, Gert van Rensburg, Cecil Uys and Jurie Uys, who made collection possible. We wish to thank veterinarian Adriaan Olivier for helping to source and collect modern ostrich eggs for the study. Izak Venter helped identify and secure places in the caravan park for our experimental season, which made it possible to conduct the pilot season. Many thanks to Dr. Carin Andersson Dahl for her invaluable contribution to collecting shellfish, helping with practical duties, and general support during the pilot season and to Professor Simon Armitage for essential assistance in the final stages of the pilot season. Dr. Jan de Vynck is thanked for his practical help in collecting the shellfish. Our samples were shipped to Norway during the pandemic by Samantha Mienties, who is our local superhero. The SapienCE leader group is thanked for their administrative support of the project.
The owl pellets, micromammal fauna, and ostrich eggshells used in the study were sourced from modern contexts, and no permits were needed to use this material. Permits for shellfish collection were obtained as per current regulations in South Africa.
The experimental season was supported by the Research Council of Norway through its Centres of Excellence funding scheme, SFF Centre for Early Sapiens Behaviour (SapienCE), project number 262618. Any opinion, finding, and conclusion or recommendation expressed in this material is that of the authors, and the funding bodies do not accept any liability in this regard, nor do the opinions, findings, conclusions or recommendations in this material necessarily represent the views of the funding bodies.
About Authors:
Silje Bentsen1,2, Magnus Haaland3,4, Jovana Milic3, Turid Hillestad Nel3, Ole F. Unhammer3, Elizabeth Velliky3
1 Nordland County, Bodø, Norway
2 School of Geography, Archaeology and Environmental Studies, University of the Witwatersrand, Johannesburg, South Africa
3 SFF Centre for Early Sapiens Behaviour, University of Bergen, Bergen, Norway
4 Institute for Archaeological Sciences, University of Tübingen, Tübingen, Germany
Bibliography
Aldeias, V., Dibble, H. L., Sandgathe, D., Goldberg, P. and Mcpherron, S. J. P., 2016. How heat alters underlying deposits and implications for archaeological fire features: A controlled experiment. Journal of Archaeological Science, 67, pp. 64-79.
Aldeias, V., Gur-Arieh, S., Maria, R., Monteiro, P. and Cura, P., 2019. Shell we cook it? An experimental approach to the microarchaeological record of shellfish roasting. Archaeological and Anthropological Sciences, 11, pp. 1-19.
Bentsen, S. E., 2012. Size matters: Preliminary results from an experimental approach to interpret Middle Stone Age hearths. Quaternary International, 270, pp. 95-102.
Bentsen, S. E., 2013. Controlling the heat: An experimental approach to Middle Stone Age pyrotechnology. South African Archaeological Bulletin, 68, pp. 137-145.
Bentsen, S. E., 2014. By the campfire. Pyrotechnology and Middle Stone Age hearths at Sibudu. Unpublished Ph.D thesis, University of the Witwatersrand.
Bentsen, S. E., 2020. Fire, Experimental archaeology at SapienCE [Blog] Available at < https://fire.w.uib.no/ > [Accessed 14.10.2021]
Bentsen, S. E., 2021. Beautiful and like a Rainbow: Experimenting with True Colours and Heat-Affected Ostrich Eggshell. [Online video presentation] Available at < https://youtu.be/3D4f6Y2qR0c > [Accessed 14.10.2021]
Berna, F. and Goldberg, P., 2007. Assessing Paleolithic pyrotechnology and associated hominin behavior in Israel. Israel Journal of Earth Sciences, 56, pp. 107-121.
Haaland, M. M., 2021. Investigating the Effect of Systematic Hearth Maintenance (Ash Removal) on the Size, Morphology and Microstratigraphy of Long-Duration Fires. [Online video presentation] Available at < https://youtu.be/D3HhmEHo80I > [Accessed 14.10.2021]
Hoare, S., 2020. Assessing the function of Palaeolithic hearths: Experiments on intensity of luminosity and radiative heat outputs from different fuel sources. Journal of Paleolithic Archaeology, 3 pp. 531-559
Karkanas, P., 2021. All about wood ash: Long term fire experiments reveal unknown aspects of the formation and preservation of ash with critical implications on the emergence and use of fire in the past. Journal of Archaeological Science, 135, p. 105476.
Mallol, C., Hernández, C. M., Cabanes, D., Machado, J., Sistiaga, A., Pérez, L. and Galván, B., 2013a. Human actions performed on simple combustion structures: An experimental approach to the study of Middle Palaeolithic fire. Quarternary International, 315, pp. 3-15.Mallol, C., Hernández, C. M., Cabanes, D., Sistiaga, A., Machado, J., Rodríguez, Á., Pérez, L. and Galván, B., 2013b. The black layer of Middle Palaeolithic combustion structures. Interpretation and archaeostratigraphic implications. Journal of Archaeological Science, 40, pp. 2515-2537.
Mallol, C., Marlowe, F. W., Wood, B. M. and Porter, C. C., 2007. Earth, wind, and fire: Ethnoarchaeological signals of Hadza fires. Journal of Archaeological Science, 34, pp. 2035-2052.
Mccauley, B., Collard, M. and Sandgathe, D., 2020. A cross-cultural survey of on-site fire use by recent hunter-gatherers: Implications for research on Palaeolithic pyrotechnology. Journal of Paleolithic Archaeology, 3, pp. 566-584.
Meincken, M., 2009. A braai-wood index: Everyday science. Quest, 5, pp. 26-27.
Mentzer, S. M,. 2014. Microarchaeological approaches to the identification and interpretation of combustion features in prehistoric archaeological sites. Journal of Archaeological Method and Theory, 21, pp. 616-668.
Miller, C. E. and Sievers, C., 2012. An experimental micromorphological investigation of bedding construction in the Middle Stone Age of Sibudu, South Africa. Journal of Archaeological Science, 39, pp. 3039-3051.
Munalula, F. and Meincken, M., 2009. An evaluation of South African fuelwood with regards to calorific value and environmental impact. Biomass and Bioenergy, 33, pp. 415-420.
Nel, T. H. and Henshilwood, C. S., 2016. The small mammal sequence from the c. 76–72 ka Still Bay levels at Blombos Cave, South Africa–taphonomic and palaeoecological implications for human behaviour. PLoS ONE, 11, p. e0159817.
Nel, T. H., Wurz, S. and Henshilwood, C. S., 2018. Small mammals from Marine Isotope Stage 5 at Klasies River, South Africa–reconstructing the local palaeoenvironment. Quaternary International, 471, pp. 6-20.
Nel, T. H., 2021. Will it burn? Micromammal Bone in Four Fire Experiments. [Online video presentation] Available at < https://youtu.be/mQf4I9Su5wA > [Accessed 14.10.2021]
Outram, A. K., 2008. Introduction to experimental archaeology. World Archaeology, 40, pp. 1-6.
Palgrave, K. C., 1977. Trees of Southern Africa. Cape Town and Johannesburg: C. Struik Publishers.
Post, J., Kleinjan, C., Hoffmann, J. and Impson, F., 2010. Biological control of Acacia cyclops in South Africa: The fundamental and realized host range of Dasineura dielsi (diptera: Cecidomyiidae). Biological Control, 53, pp. 68-75.
Schmidt, P., Porraz, G., Bellot-Gurlet, L., February, E., Ligouis, B., Paris, C., Texier, P.-J., Parkington, J. E., Miller, C. E., Nickel, K. G. and Conard, N. J., 2015. A previously undescribed organic residue sheds light on heat treatment in the Middle Stone Age. Journal of Human Evolution, 85, pp. 22-34.
Shahack-Gross, R., Berna, F., Karkanas, P., Lemorini, C., Gopher, A. and Barkai, R., 2014. Evidence for the repeated use of a central hearth at Middle Pleistocene (300 ky ago) Qesem Cave, Israel. Journal of Archaeological Science, 44, pp. 12-21.
Sievers, C. and Wadley, L., 2008. Going underground: Experimental carbonization of fruiting structures under hearths. Journal of Archaeological Science, 35, pp. 2909-2917.
Smit, H. C. and Meincken, M., 2012. Time/temperature combustion profiles of various wood-based biofuels. Biorefinery, 39, pp. 317-323.
Tietema, T., Ditlhogo, M., Tibone, C. and Mathalaza, N., 1991. Characteristics of eight firewood species of Botswana. Biomass and Bioenergy, 1, pp. 41-46.
Unhammer, O. F. and Armitage, S. J., 2021. 4-Dimensional Recording of Fire-related Experiments. [Online video presentation ] Available at: < https://youtu.be/8_WRpljH2vY > [Accessed 14.10.2021]
Vallverdú, J., Alonso, S., Bargalló, A., Bartrolí, R., Campeny, G., Carrancho, Á., Expósito, I., Fontanals, M., Gabucio, J., Gómez, B., Prats, J. M., Sañudo, P., Solé, À., Vilalta, J. and Carbonell, E., 2012. Combustion structures of archaeological Level o and Mousterian activity areas with use of fire at the Abric Romaní rockshelter (ne iberian peninsula). Quaternary International, 247, pp. 313-324.
Wadley, L., 2006. The use of space in the late Middle Stone Age of Rose Cottage Cave, South Africa: was there a shift to modern behavior?, in: E. Hoversand and S.L. Kuhn (eds.) Transitions before the transition evolution and stability in the Middle Palaeolithic and Middle Stone Age.. New York: Springer. pp. 279-294.
Wadley, L. and Prinsloo, L. C., 2014. Experimental heat treatment of silcrete implies analogical reasoning in the Middle Stone Age. Journal of Human Evolution, 70, pp. 49-60.