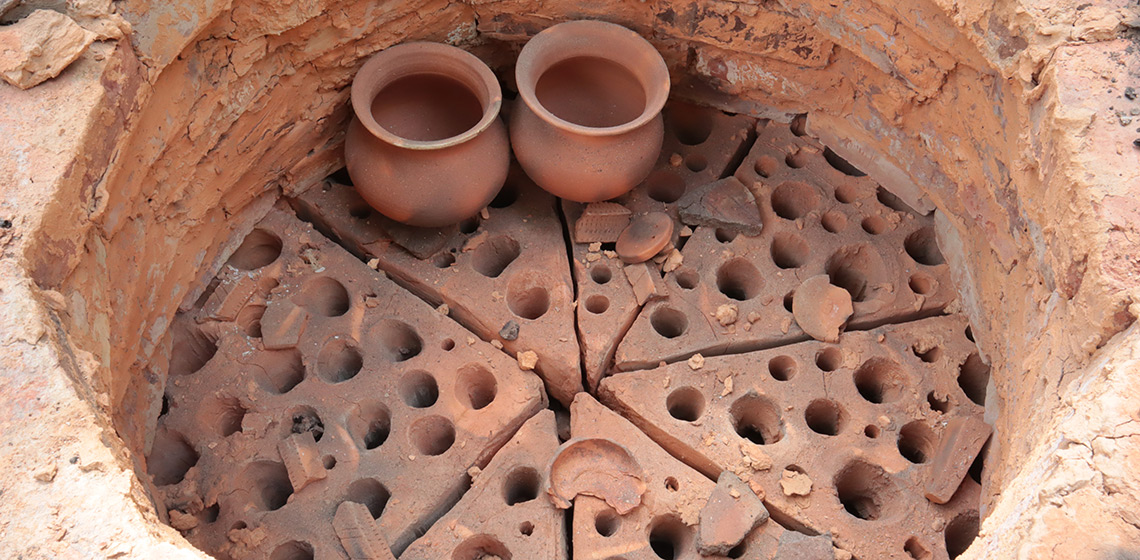
This article presents an experimental archaeology project that aimed to reproduce the Hellenistic Greek pottery production process. The project's main research questions were focused on understanding how locally available raw materials and climatic conditions influenced the production process and how the process created social networks with the local community. Based on archaeological data from the Hellenistic site of Sant'Angelo Vecchio in the territory of Metaponto and ethnographic evidence from rural potters in Spain, the project replicated all stages of the pottery-making process, including clay collection, wheel-throwing, kiln building, and firing. The project's successful outcome demonstrated that the combination of archaeology, ethnography, and personal skills can yield valuable insights into ancient pottery production processes. Furthermore, the experiment confirmed that pottery production was part of a larger social network that facilitated the exchange of raw materials and ceramic products between potters and their communities.
de entre todos el primero
porque en la industria del barro
Dios fue el primer alfarero
y el hombre el primer cacharro.
[Noble and valiant craft
the first of all
because in the industry of clay
God was the first potter
and the human being the first pot.]
(Traditional poem of the Spanish potters)
Introduction
This experimental project aimed to reproduce the Hellenistic (fourth-third century BC) Greek pottery production process. The project was conducted by the authors, Francesca Tomei, PhD graduate in Archaeology at the University of Liverpool, and Juan Ignacio Jimenez Rivero, a ceramist specializing in replicating ancient pottery technology, who frequently collaborates with the Department of Classics, Ancient History, Archaeology and Egyptology, University of Manchester, on ceramic experimental archaeology projects and activities. The project was funded by the Arts and Humanities Research Council (AHRC) North Western Consortium Doctoral Training Partnership (NWCDTP) Fieldwork Fund for doctoral students.
We intended to address two main research questions:
1. How did locally available raw materials such as clay, temper, and fuel, as well as the local climatic conditions, impact the decision-making process, the production process, and the successful firing of ancient Greek pottery?
2. How can we improve our understanding of the ancient Greek pottery production process in a suburban context, including the social networks created with the local community?
The experiment took place on Juan Ignacio's private property in Whalley Range, southwestern Manchester. Although we had originally planned to complete the project in one year, working mainly in the summer months when outdoor activities are possible in the British weather, the COVID-19 outbreak and subsequent travel restrictions delayed us by a year and a half. This article provides a chronological description of each stage of the operational process, starting with the methodological approach that combines archaeological data and ethnographic information and concluding with the firing of the pottery, as well as a discussion of the results and observations about the experimental process.
Stage 1: Research and Preparation of the Experimental Project
This experimental project is based on thorough research on kiln technology in the ancient Greek world through the study of the archaeological evidence from excavations in Greece and Southern Italy from the Archaic (sixth century BC) to the Hellenistic period (third century BC) (Hasaki, 2002; Tomei, 2017). During this chronological period, the Greek artisans used vertical or updraft kilns, which are pyrotechnological structures with two chambers separated by a perforated floor and covered by a dome. In such kilns, the draft allows the heat to move upwards thanks to the stoking channel at the bottom and the chimney at the top of the dome (Hasaki, 2002, p. 72). These kilns have many advantages over pit firing, including better thermal insulation, more economical consumption of fuel, and larger capacity; in addition, they can reach a maximum temperature of ca. 1100°C, limiting the risks of overfiring the pottery (Hasaki, 2002, pp. 72–73). Another and most important advantage is that double chamber kilns protect vases from direct contact with fire, and so reduce the risk of breaks and cracks. We focused on the rural workshop of Sant'Angelo Vecchio in the territory of the Greek city of Metaponto, in southern Italy, as a specific case study and archaeological reference, especially for the pottery assemblage (Silvestrelli and Edlund-Berry, 2016). The first phase of the production site belong to the late fourth-early third century BC and it had three small- to medium-sized kilns producing terracotta votive plaques, utilitarian plain and coarse wares, table wares and loom weights (Silvestrelli, 2016). It was a context presenting a wide variety of pottery and ceramic products for everyday-life use in a rural settlement, allowing us to experiment with different shapes and dimensions without complicated painted figures requiring high painting pottery skills that need many years of training and apprenticeship.
Besides the archaeological remains, the Penteskouphia plaques helped to reconstruct the general appearance of the ancient Greek kilns and the tools used by the potters. It is a large group (ca. 1000 pieces) of votive terracotta plaques (pinakes) from a sanctuary located south-west of Corinth and they date back to the first half of the sixth century BC according to the iconographical style (Hasaki, 2022, p. 2). About 100 pinakes depict potters at work, digging clay, wheel-throwing and decorating vessels and firing the kilns. They have been an important iconographical source to build the kiln as the ancient Greek potters did, especially the firing chamber and the dome. In particular, some pinakes (e.g., Plaque Penteskouphia MNB2858) show an opening in the firing chamber interpreted as a loading door (Hasaki, 2022, pp. 101 and 106), but most likely it was an opening to take out test pieces to check the firing since it seems too small to put in the vessels and stack them properly (See Figure 1). Thus, we planned an opening to check the state of the black gloss. In order to be as much accurate as possible, we made the replicas of two tools depicted in some plaques: a T-shaped rod (Hasaki, 2022, p. 89 Fig. 4.4, p. 90 Fig. 4.5, p. 118 Fig. 4.32), probably used to manage the fire and the ashes, and a hooked tool (Hasaki, 2022, p. 123 Fig. 4.37), most likely to take out the samples to check the gloss melting (See Figure 2). Indeed, we have observed that a similar hooked tool has been used for such purpose until recently in many traditional potteries in Spain, for instance, in Naval in the province of Huesca (E. Monesma, 1991) and in Cuenca (El Primer Cacharro, 2018). The material of the tools is not known archaeologically but since they had to be in touch with fire, we agreed to ask a smith to make them with recycled wrought iron.
The creation of a database of ancient Greek kilns for the author’s MA dissertation (Tomei, 2017) in addition to Hasaki’s catalogue (Hasaki, 2002) allowed us to extrapolate the most common shape and the average dimensions of medium-capacity pottery kilns based on the archaeological evidence. Therefore, we planned a one metre inner diameter kiln with a circular combustion chamber with a central pillar to support the perforated floor (Type Ia: Cuomo Di Caprio, 1971; Hasaki, 2002) The stoking channel is 0.6 m long and wide. The permanent part of the firing chamber is 0.5 m high, and the temporary dome is 0.55 m, reaching a total height of 1.05 m. The shape and size of the dome, certainly, may have depended on the amount of pottery to fire and ancient potters may have adapted the dome to the specific needs of each firing. Since they do not survive in the archaeological record, the height of the firing chamber and the dome are unknown. We adapted that dimension to the load we were going to fire, according to ethnoarchaeology. Ethnography has been an essential source of knowledge as it has helped us to recreate the different phases of the ancient Greek production process, including how to build the kiln and manage the firing. The ethnographical research that supported this project was mainly focused on traditional potters from the rural Iberian peninsula (Valladolid, Castilla and Galicia) using preindustrial methods inherited from the Graeco-Roman and Arabian pottery traditions (Sempere, 1982; González, 1989; Seseña, 1997; Castellote Herrero, 2006; García Alén, 2008).
Stage 2: Selection of clay and its processing
According to ethnographic sources, clay is preferably collected in the proximity of the workplace to save time and energy as clay is needed in great quantity and more frequently than slips or paints (Matson, 1972; Arnold, 1985; Gosselain and Smith, 2005; Tomei, 2022a). Luckily, suitable plastic clay is naturally abundant in the Whalley Range area and we could obtain it from the diggings made in public and housing works. Its colour varies between grey and brown due to the filtration of black particles from the upper fertile soil. However, it is quite a pure clay without many limestone inclusions, nevertheless, it must be carefully filtered to remove them and avoid damage in the vessels.
Unlike the traditional Spanish potters, who used two large pools to dissolve, filter and set the clay to dry, and also due to space limitations and humid climate, we soaked the natural clay in buckets during the winter months to allow it to soften and improve its plasticity. From the beginning of spring, we started filtering clay, which is a laborious process consisting in squeezing the clay and sieving it through a plastic mesh ca. 1.5 mm wide and again through a finer one of less than 1 mm. This procedure ensures that large pebbles and other impurities are removed from the clay fabric. The resulting finer mud settles for several weeks or even months before the clear water on the top is skimmed off.
As pots must stand tensions and changes during firing, we added finely sieved quartz sand to increase clay porosity and make it more resistant to expansion and contraction. Porosity is particularly important when firing pottery in wood-fed kilns because water needs an easy escape in form of steam when the pots warm up to 200°C. Otherwise, pots would easily explode and spoil the potters' work as a result. Losing a bulk of vessels was a cost that a rural ancient potter could not afford.
Stage 3: The Making of the Mudbricks and the Perforated Floor
In most ancient Greek kilns, the combustion chamber was dug into the soil and the walls were covered with mudbricks and clay (Hasaki, 2002). We modelled the handmade mudbricks following the traditional techniques from Valladolid in Northern Castile (González, 1989): we mixed natural clay with sand to make the bricks more refractory to high temperatures and then we packed the tempered clay into a wooden frame (See Figure 3), whose dimensions (9x20 cm) are based on the few remains of mudbricks in the archaeological record (Hasaki, 2002). In one day, we made ca. 100 bricks and laid them outdoors to sun dry for a couple of sunny days (See Figure 4). Since there is evidence of the use of cereal chaff and straws as temper in the production of bricks for the structure of the kilns (Metaponto chora, Sant’Angelo Vecchio: (Costantini and Pica, 2016; Silvestrelli and Edlund-Berry, 2016), we also made adobe bricks by tempering clay with straws of lawn grass clippings - we did not need to chop them because they were thin and flexible - gathered from the public park nearby (See Figure 5). Adobe bricks are important for their insulating properties, and we planned to use them for building the temporary dome and the chimney. Abundant ethnographic evidence confirms the use of adobe bricks as a kiln building material across Spain, such as in Guadalajara (Castellote Herrero, 2006) and in Jiménez de Jamuz in León, where we assisted in a firing in a Moorish kiln in August 2022.
The intention was to build the central pillar and most of the kiln’s permanent structure with raw handmade bricks. However, we realised that the process cost a significant amount of time and energy, and the extreme humidity of the soil would have caused damage to the structure as it would make the raw bricks crumble. Therefore, fired Victorian bricks were a valuable and accessible resource from building sites in the neighbourhood. Their manufacturing process did not differ much from the ancient one, they had been fired in charcoal or coal kilns and they have refractory properties (Lynch, 2022). Consequently, we decided to use Victorian bricks to build the permanent structure, including the firing chamber and the central pillar supporting the perforated floor. In contrast, the less robust handmade bricks and adobe bricks were used to build part of the stoking channel and the dome.
The second most challenging element was the making of the perforated floor. The perforated floor, or eschara in Greek, is an essential feature of the updraft kiln and it separates the firing chamber from the combustion chamber, prevents the pots from direct contact with the flames and allows less heat to reach the firing chamber (Hasaki, 2002). The perforated floor was rarely found in situ, but blocks collapsed inside the combustion chamber or in other parts of the archaeological deposit – e.g., Prinias (Kiln 2/B) in Crete (Rizza, et al., 1992; Hasaki, 2002) or Sindos in Macedonia (Hasaki, 2002, p. 359) - suggested that the average thickness was between 0.07 and 0.20 m. The diameter of the vent holes usually varied between 0.06 and 0.10 m (Hasaki, 2002, p. 83). The perforated floors from modern traditional pottery kilns in Margarites (Crete) were 0.10-0.15 m thick and the diameter of the holes was similar to the ancient ones (personal observation, 2019). Based on this information, we designed a perforated floor with a 1 m diameter, the same as the combustion chamber, and 0.10 m thickness. We considered a 10% shrinkage rate after drying, so we added 0.10 m to the diameter and 0.02 m to the thickness to avoid the risk to obtain a perforated floor smaller than the combustion chamber’s diameter. We decided to build it by making six portions averaging an angle ca. 45° each after considering practical issues since lifting and placing the entire thick platform would have been heavy and the portions would dry out more quickly. By doing this, we would also prevent some possible cracks during the drying process. On the other hand, fractures during firing would only be limited to the sections affected and not to the whole platform. In addition, there is evidence of this technique being applied in Roman pottery kilns (Rhodes, 1986, p. 16, fig. 18; Papadopoulos and Schilling, 2003, p. 208, fig. 3.14).
Our perforated floor was made with finely sieved local clay mixed with 30% of coarse building sand rich in quartz to produce a refractory clay body that could withstand thermal expansions and contractions due to heat and flame contact. We carefully kneaded big clay loaves to remove air pockets and, after having dampened them, we modelled each portion by sticking them together starting from the centre towards the external part. We placed a wooden stick at the centre of the working table and used a rotating cord to measure the radius of each module and, thus, the diameter of the entire floor (See Figure 6). We smoothed the surface of each portion and made the vent holes with a rod. The holes were between ca. 0.06 m and 0.08 m on the external edge to achieve a more homogeneous temperature across the firing chamber (See Figure 7). The holes, besides allowing the draft and the heat to reach the firing chamber, facilitated the drying of the clay body and helped avoid cracks and explosions in the platform during the firing.
Making the perforated floor was a very laborious and time-consuming task since it required an average of three hours per day to mix and knead sand and clay and one hour to shape each section, for a total of seven days to complete it. Moreover, it took up to six months to completely dry them out, due to the thickness of the platform, the humid Manchester climate and the dump cellar where we stored them (See Figure 8).
Stage 4: Wheel-throwing the pottery
Amongst Sant’Angelo Vecchio’s Early Hellenistic assemblage, we selected a representative sample of vessels for everyday domestic use, such as eating, cooking, serving, drinking and storing food and liquids. Before wheel-throwing the pots with a traditional Spanish kick wheel (See Figure 9), we carefully studied the published archaeological drawings by sketching them on paper to reflect on their shapes and dimensions (Silvestrelli and Edlund-Berry, 2016). To each vase, we added a 10% size to compensate for the average shrinkage of our clay after drying and firing.
According to the use of the vessels, we chose the kind and percentage of temper to add to the clay, as the ancient potters used to do following the tradition that they learned within their community (Wendrich, 2013). For instance, for pots used for cooking food on the stove on in the oven, we added ca. 1/3 of sieved sand to the clay volume to improve their refractory quality. For vessels made for drinking or pouring liquids, we included less sand in the fabric, only ca. 1/5 of the clay amount which is the necessary proportion to allow vases to resist thermal shocks in the kiln.
Since we selected some Black Gloss wares, we tried to achieve such a surface treatment using a red-coloured slip, similar to sigillata, which will turn black during the reduction phase of the firing. We reproduced the slip following a modern formula (Kip O'Krongly, 2015: https://youtu.be/s_1fhFL6zgw) consisting of 1200 g of sieved fine red clay, 2.8 litres of water and 6.5 ml of sodium silicate. We let the solution rest for 72 hours, then we siphoned it and reduced it to a dense slip, which we later applied to the pots on several coatings by brushing or immersion, according to the archaeological descriptions in (Noble, 1988; Cavallo, 2016; Conoci, 2016) (See Figure 10).
Stage 5: Building the Kiln
We adapted an already existing firing pit used for Neolithic pottery firings to make the combustion chamber by widening it up to 1 m diameter and deepening it up to 0.6 m, based on the archaeological evidence (Hasaki, 2002). Whilst digging the bottom of the combustion chamber, we reached a level of silty clay, of which we stored a good quantity to make the cocciopesto mix to insulate the walls and the floor of the combustion chamber from soil humidity and flooding. Then we dug the stoking channel ca. 0.6 m long and wide, leaving a gentle slope towards the firing pit to ease the entrance of the air to feed the fire. Since the garden is surrounded by walls, the stoking channel is oriented to the east facing the entrance to the garden to get the advantage of the winds blowing from that direction (See Figure 11).
Following the archaeological evidence, we built an arch at the mouth of the combustion chamber as a connection with the stoking channel, sitting on two columns of Victorian bricks. The space between the combustion chamber floor and the arch is 0.62 cm, enough to let the draft go inside. We designed a wooden arch-shaped frame on which we placed two rows of bricks, vertically on the first row with a central brick in the vortex as a keystone and horizontally on the second one. The gaps between the bricks were filled with pebbles to keep the angles and allow the bricks to hold against each other, enhancing the stability of the arch (See Figure 12). Once the bricks were thoroughly arranged, we carefully pushed out the frame and reinforced it with more pebbles filling the remaining gaps. The structure successfully stood up thanks to the force produced by its own weight (See Figure 13).
Afterwards, we built a provisional central pillar to support the perforated floor and tested its stability by placing two portions of the floor on top of it. However, it proved to be not safely stable. To solve that structural weakness, we covered the whole perimeter of the combustion chamber with Victorian bricks to make a circular wall to safely support the outer part of all the portions of the perforated floor, while all the central angles would rest on the central pillar. That circular wall started at the base of the combustion chamber, and it stood above the ground as high as the top of the stoking channel arch to provide a horizontal sit for the perforated floor portions. The wall section underground, at the level of the combustion chamber, was laid using the technique called ‘Hit and Miss Brickwork’. The resulting gaps were filled with a cocciopesto lining (See Figure 14). Cocciopesto is a mixture of clay, finely mashed bricks- using a spade- and water, which is necessary to make the mixture softer and more manageable. The mashed bricks make the mixture somewhat impermeable and refractory. We applied a 0.02-0.03 m layer of cocciopesto also on the combustion chamber floor to possibly avoid flooding, which may happen in case of heavy rains (See Figure 15).
The central pillar to support the perforated floor in Type Ia Greek kilns had roughly a circular or rectangular shape and it was made of mudbricks or clay mortar mixed with broken sherds, tiles and stones (Hasaki, 2002; Tomei, 2017). However, the humidity of the combustion chamber and the weight of the perforated floor could enhance the risk of collapse during the pre-firing stage. Therefore, we agreed to use well-fired Victorian bricks to make a more stable and resistant pillar. We found the right position of the pillar inside the combustion chamber using cardboard pieces tracing the perforated floor’s portions as they were too heavy to handle. We placed them on the top of the column and found the most stable position both for the pillar and the perforated floor sections (See Figure 16). We did not use any clay mortar to stick together the bricks and fill the gaps, because the arrangement of the bricks and the weight of the perforated floor would make the column strong and stable.
After completing the combustion chamber, we built the structures of the stocking channel. Next to the arch at the entrance of the combustion chamber, we made two small columns of bricks and along the ditch we placed two rows of bricks at the same height as the columns, filling the gaps with pressed soil. The walls along the channels are 0.6 m long. Then we built the covering of the tunnel using seven arch-shaped flat coils that we made three months before during the summer. The coils are made of unrefined clay mixed with coarse lime/free sand and mashed bricks. We used the wooden arch frame to model them to the right shape. As the tunnel must be 0.6 m long, the coils were about 0.10 m wide considering the shrinkage rate of the clay body after drying. We positioned the coils over the brick rows and placed other Victorian bricks on both sides as counterweights (See Figure 17). Then, we stuck them together and filled the gaps with a cement made of clay mixed with water and reinforced the tunnel with two layers of adobe bricks held together with clay cement (See Figure 18). Once the structure of the stoking channel was dried out and pre-fired, it became strong and stable and did not suffer any breakage.
A couple of weeks after we completed the combustion chamber and the stoking channel, we placed the perforated floor following the pattern with the cardboard that we previously agreed as it was the most stable. We also filled with clay the gaps between the floor and the combustion chamber and the arch to avoid heat losses.
Afterwards, we pre-fired the kiln to just above 400°C to dry out the combustion chamber and the perforated floor as well as to test the draft. Although temperatures around 600°C would have been necessary to fire the perforated floor, we could not reach them due to the lack of the dome that retains the heat, so we considered 400°C a prudent and adequate temperature for this initial test without risking fuel waste and cracks in the kiln’s structure. We spent the first three hours to reach 100°C because water trapped within the thick floor modules should be released gently to avoid fractures. We happily succeeded with this challenge as the perforated floor withstood the thermal change without any damage. We also found that the kiln’s draft was excellent since even the mildest winds conducted the hot gases from the stoking channel through the vent holes of the platform (See Figure 19).
After the pre-firing, we covered the kiln structure for the winter with three layers of tarpaulin fixed to the ground with heavy bricks all around1 . Nevertheless, the following May we found out that soil humidity caused condensation that made the perforated floor humid and crumbly again. Therefore, we let it dry under the sun for three weeks and pre-fired it again to dry it out completely and make it resistant to the firing. After that, we built the firing chamber. First, we arranged an additional outer protection for the combustion chamber with two rows of Victorian bricks held together with natural unrefined clay. Starting from the perforated floor, we placed six rows of bricks up to 0.55 m high and clay cement and filled the gaps with natural clay to ensure thermal insulation (See Figure 20). Like in some Penteskouphia plaques (see Figure 1), on the northern side of the firing chamber (See Figure 11), we made a small test window to monitor the firing process and the vitrification of the sigillata slip. The window could be closed with removable bricks.
Stage 6: Stacking the kiln and building the dome
We stacked the kiln following the ethnographic evidence from different regions in Spain (González, 1989; Castellote Herrero, 2006; García Alén, 2008) and our own experience with Neolithic and Bronze Age pit firing as well as the gas kiln. We laid the larger cooking vessels (casseroles, pans, jars) at the base of the load with smaller coarse wares at the centre (casseroles, jugs) because they are made of more refractory fabric and could stand against contact with the flames. These vessels rested on small flat sherds to not to obstruct the vent holes of the perforated floor and let the flames spread evenly without obstacles. Above them, we placed finer plain and painted bowls and cups. The basins on top of the load were placed upside down to retain the heat of the ascending gases (See Figures 21 and 22). We carefully arranged each pot halfway above two vessels to create a frame like a brick wall to prevent greater losses if any vase would explode during the firing.
As the pots heap was growing, we built the temporary dome as high as the kiln load required using the handmade raw bricks cemented with natural clay. We completed the dome with a chimney ca. 0.30 m high and wide (inner diameter). The final shape of the dome did not look like the semi-spherical ones depicted on some Penteskouphia pinakes but it was the result of the specific pottery load that we had to fire. Furthermore, the kilns in the pinakes do not always show the same size or shape, so this experience may confirm the assumption posed in Stage 1 that the shape and size of the dome could vary according to the specific needs of each firing.
Stage 7: The firing
The provision of fuel was another essential part of the experiment in preparation to the kiln firing. The residential area of Whalley Range has many private and public gardens and parks where abundant branches and sticks from local trees and woody plants can be gathered for free, as well as wooden pallets and carpentry leftovers. For this firing, we stored many branch cuttings and planks collected in the surrounding area at different times. Indeed, the collection of fuel shows how traditional pottery production can be part of the local community network, which is made of human relations, favour exchange, mutual aid, and trade in kind. In this way, people get rid of garden pruning and scraps by giving them for free to the potter who needs them to carry on his activity without additional costs. In the rural areas, the use of agricultural waste is documented in antiquity and modern times in the Mediterranean, and the most common were olive and vine branches taken after the seasonal pruning, olive pomace, in addition to sticks and branches from the Mediterranean maquis (Foxhall, 1998; Rowan, 2015; Tomei, 2022b).
Summer is the most suitable time of the year for firing pottery in the kiln because the weather is warmer and there is less chance of rain during the process, which can affect the outcome (Arnold, 1985). Therefore, we agreed to plan it for mid-July. First, we placed five thermocouples to monitor the temperatures in the firing chamber throughout the process: four of them on each side of the firing chamber just above the perforated floor and the fifth one at the junction between the firing chamber and the dome on the southern side of the kiln (See Figure 23). The firing team was composed of five people to help gather and add fuel, prepare food for everybody, and monitor the temperatures every five minutes to make sure they increased gently. In addition to the thermocouples, we monitored firing stages and the melting of the sigillata slip using a traditional method. It consists of taking a sample with an iron hook (see Stage 1) through the test window made on the firing chamber. Firing has always been the most delicate stage of the pottery production sequence, as the numerous ancient Greek depictions of kilns with apotropaic objects or rituals against disasters demonstrate (Noble, 1988; Hasaki, 2002; Hasaki, Tzonou and Herbst, 2022). In the Galician town of Portomourisco, the firing was monitored by a specific highly skilled person called “el enfornador”, who took care of the entire operation to achieve a successful outcome (García Alén, 2008).
We planned the firing based on the ethnographic evidence of rural Spanish potters (García Alén, 2008) and our experience with gas kiln and pit firing and the planned steps are displayed in Graph 1. Consequently, we cautiously started the fire with a few branches at the entrance of the stoking channel, allowing the hot smoke to gently warm up the kiln and the pots inside. In this way, the perforated floor, still raw as the hand-made bricks, would have softly fired to ceramics without risking massive breaks. We could monitor these early stages of the process also by feeling a warm and humid steam with our hands on top of the chimney, caused by the water trapped in the clay being released in the form of steam. As shown in Graph 2, we spent two hours to attain 100°C (See Figure 24).
At temperatures above 150°C, we observed that despite spreading the ashes and flames evenly, the temperature measured by the thermocouples diverged quite remarkably and indicated that the northern and western sides of the firing chamber remained colder than the others. These sides of the kiln faced abundant vegetation that kept the soil more humid and, consequently, the kiln temperature lower. Direct observation of the kiln and the surrounding environment is important for potters to achieve a successful firing. By knowing the hottest or coldest areas of the kiln, they could arrange pots and manage fuel and fire accordingly.
After three hours of prudent feeding, we reached a heat above 200°C, so we decided to add more branches and solid logs to keep a constant increase in temperature. As we proceeded, we pushed more and more red ashes with the T-shaped rod into all sides of the combustion chamber (See Figure 25). We continued operating in this way for the next four hours until we reached an average temperature of ca. 700°C. As temperatures were rising, we found that the clay cement dried out, forming many small gaps we had to cover with more clay to prevent heat loss.
Despite this, after 700°C, the temperature increase was less pronounced than before (See Graph 2) because of the poor insulation provided by the single layer of adobe and clay bricks in the dome. As observed directly in Jiménez de Jamuz in the province of León, traditional potters solved this issue by building their kilns with two or more layers of adobe bricks or stones or by setting them against a slope (Castellote Herrero, 2006; García Alén, 2008).
Nevertheless, after two more hours of intense feeding with small branches to get a vivid and intense flame, the firing reached temperatures ranging between 800°C and 900°C (See Figure 26). At this point, we opened the small test window to take a sample of a small cup and found out with great satisfaction that the sigillata vitrifying slip had already started to become black and the pots turned into an intense orange glowing, which is a sign of well-fired wares (See Figure 27). We immediately closed the window again and reduced the atmosphere by adding abundant green leaves until thick smoke came out of the chimney. After a while, when the temperature went below 750°C, we stopped adding any foliage to allow oxygen to enter the combustion chamber and allow the parts of the pots that had not been coated with sigillata slip to recover their oxidized orange colour. After this stage was completed and after 10 hours of firing, we sealed off the entrance of the kiln to slowly cool it down. Then we waited 48 hours to open it and check the outcome.
The firing was outstandingly successful as no pot broke or exploded during the process or after opening the kiln (See Figure 28). They showed no sign of misfiring, except for a few pans and casseroles than were at direct contact with the perforated floor and became over-fired as a result (See Figure 29). Nevertheless, a potter could have sold those vessels with minor flaws at a lower price. The black gloss came out dark, shiny, and uniform on most vessels, especially the open ones such as the bowls and the Ionic cups (See Figure 30). Contrarily, on some vessels the black gloss appeared uneven probably because a thicker layer of slip should have been applied with the brush. Also, the perforated floor fired well and homogeneously and resisted to the contact with the flames without any severe crack at all (See Figure 31). It can be used for other more firings without having to replace any of the modules.
Conclusions
Overall, the combination of archaeological evidence, ethnographic research on traditional Spanish potters and previous pottery-making experience resulted in a successful replication of the Hellenistic Greek pottery-making process and firing. Ethnography proved to be quite helpful in providing the practical information missing in the archaeological record, such as how to stack the pots in the kiln and manage the fire during the different firing stages.
The use of the local clay available for free in the area surrounding the workplace was entirely satisfactory for the making of good quality coarse and fine vessels, mudbricks, and adobe bricks, by adding the appropriate temper (sand, grog, straws). Similarly, gathering fuel from the neighbours proved to be a very economical and practical way as it offered mutual advantages: the former could easily get rid of the garden pruning or carpentry scrap, and the latter obtained free good fuel for the kiln in the quantity needed for one firing. In a similar way, ancient Greek potters mostly used agricultural waste as a source of fuel obtained by the farms and other rural sites in the proximity of the workshop. Indeed, the choice of the location of the pottery workshops was driven by the availability of clay within a short distance and the presence of residential or non-residential rural sites because they were part of a network of exchange of raw materials and ceramic objects (Tomei, 2022b, 2022a).
The climate, different from the Mediterranean, substantially conditioned the timing of most of the manufacturing stages and how to build some structural parts of the kiln. Since the experiment was undertaken in Manchester where the local climate is mostly humid and rainy in the summer. the outdoor tasks could only be performed in good weather, slowing down the construction of the kiln and delaying the firing. The humid climate also negatively influenced the drying of the perforated floor and the wheel-thrown pottery. In addition, the frequent heavy rains caused several flooding episodes in the firing pit, so it was necessary to coat its walls and ground with bricks and cocciopesto to make the combustion chamber more impermeable and insulated.
Lastly, we could confirm that practice is important to gain the skills necessary to accomplish the production process and to solve the problems that we might face. For example, we learned the best temper for each kind of fabric and further use of the vessel and the best fuel for each stage of the firing.
This experiment can lead to several possible further outcomes. The kiln can be a source of educational activities for a British audience to understand the meaning and technological context of the Roman kilns found throughout England since they are a development of Greek technology. Figurine, pot making, and decoration can add genuine excitement to experimental archaeology activities. Furthermore, the wide variety of Late Hellenistic household pots can be used for teaching everyday life and cooking practices in Ancient Greece and Magna Graecia.
In addition, without the wisdom and knowledge of traditional rural potters who made the most beautiful pots until recent times with so little recognition, this experiment would have not been possible. Let this kiln and its pots be a humble tribute to all the rural potters who still survive in the world.
Acknowledgements
We wish to thank Dr Ina Berg of the University of Manchester for giving us the idea for this project and putting us in touch. Her support throughout this big project has always been hugely appreciated. We also express our gratitude to the AHRC NWCDTP for funding this experimental archaeology project. Lastly, but not least importantly, we thank Ignacio’s family for making available part of their garden to build the kiln, Mark for manufacturing the T- shaped rod and hooked tool, and all the friends who took part and helped us during the firing.
- 1We used this structure to protect the working area from rain, temperature change, wind, pets, animals as well as from theft and vandalism over the long periods.
Bibliography
Arnold, D.E., 1985. Ceramic theory and cultural process. Cambridge University Press (New studies in archaeology).
Ayuntamento de Cuenca, 2018. El Primer Cacharro. [Video Online]. Available at: < https://www.youtube.com/watch?v=PRr0pqAPseY&t=2817s > [Accessed 25 June 2023].
Castellote Herrero, E., 2006. Alfarería de Guadalajara: una guía para conocerla. Guadalajara: AACHE Ediciones de Guadalajara (Tierra de Guadalajara, 62).
Cavallo, A., 2016. Banded Ware. In: F. Silvestrelli and I.E.M. Edlund-Berry, ed. 2016. The Chora of Metaponto 6: A Greek Settlement at Sant’Angelo Vecchio. University of Texas Press, pp. 279–306.
Conoci, E., 2016. Black-gloss Ware and Lamps. In; in F. Silvestrelli and I.E.M. Edlund-Berry ed. 2016. The Chora of Metaponto 6: A Greek Settlement at Sant’Angelo Vecchio. University of Texas Press, pp. 221–252.
Costantini, L. and Pica, F., 2016. Charred Plant Remains and Plant Impressions in Fired Clay Fragments from Sant’Angelo Vecchio, In: F. Silvestrelli and I.E.M. Edlund-Berry ed. 2016. The Chora of Metaponto 6: A Greek Settlement at Sant’Angelo Vecchio. University of Texas Press, pp. 173–180.
Cuomo Di Caprio, N., 1971. Proposta di classificazione delle fornaci per ceramica e laterizi nell’area italiana dalla preistoria a tutta l’epoca romana. Sibrium, (11), pp. 371–464.
Foxhall, L.,1998. Snapping up the Unconsidered Trifles: the Use of Agricultural Residues in Ancient Greek and Roman Farming. Environmental Archaeology, 1(1), pp. 35–40. Available at: < https://doi.org/10.1179/env.1996.1.1.35 >.
García Alén, L., 2008. La alfarería de Galicia: catalogación arqueológica y artística de Galicia del Museo de Pontevedra. Ed. facsim. La Coruña: Fundación Pedro Barrié de la Maza.
González, P., 1989. Cerámica preindustrial en la provincia de Valladolid. Valladolid: Caja de Ahorros Provincial de Valladolid.
Gosselain, O.P. and Smith, A.L., 2005. The source: Clay selection and processing practices in sub-Saharan Africa. In: A. Livingstone-Smith, D. Bosquet, and R. Martineau ed. 2005. Pottery manufacturing processes: reconstitution and interpretation. International Congress of Prehistoric and Protohistoric Sciences, Oxford: BAR Publishing (BAR international series, 1349), pp. 33–47.
Hasaki, E., 2002. Ceramic Kilns in Ancient Greece: technology and organization of ceramic workshops. PhD. University of Cincinnati.
Hasaki, E., 2022. Potters at work in ancient Corinth: industry, religion, and the Penteskouphia pinakes. Princeton, New Jersey: American School of Classical Studies at Athens (Hesperia. Supplement, 51).
Lynch, G.C.J., 2022. Brickwork: Historic Development, Decay, Conservation and Repair. [Online]. Available at: < https://www.buildingconservation.com/articles/brick/brickwork.html > [Accessed 6 July 2022].
Matson, F.R., 1972. Ceramic Studies. In: W.A. McDonald and G.R. jr Rapp ed. 1972. The Minnesota Messenia Expedition: Reconstructing a Bronze Age Regional Environment. Minneapolis, MN: The University of Minnesota Press, pp. 200–224.
Monesma, E., 1991. Documentary Film On The Traditional Pottery In Naval, Huesca. [Video Online]. Available at: < https://www.youtube.com/watch?v=XbnrXBMvzQQ > [Accessed 25 June 2023]
Noble, J.V., 1988. The techniques of painted Attic pottery. London: Thames and Hudson.
O'Krongly, K., 2015. How To Make Terra Sigillata. [Video Online]. Available at: < https://youtu.be/s_1fhFL6zgw > [Accessed 25 June 2023].
Papadopoulos, J.K. and Schilling, M.R., 2003. Ceramicus Redivivus: The Early Iron Age Potters’ Field in the Area of the Classical Athenian Agora. Hesperia Supplements, 31, pp. iii–370. Available at: < https://doi.org/10.2307/1354054 >
Rhodes, D., 1986. Kilns. Design, Construction and Operation. Radnor, PA: Pitman Publishing.
Rizza, G., Palermo, D. and Tomasello, F., 1992. Mandra di Gipari. Una Officina Protoarcaica di Vasai nel Territorio di Priniàs. Catania: Università di Catania, Istituto di Archeologia, Consiglio Nazionale delle Ricerche, Centro di Studi per l’Archeologia Greca (Studi e Materiali di Archeologia Greca - Priniàs).
Rowan, E., 2015. Olive Oil Pressing Waste as a Fuel Source in Antiquity. American Journal of Archaeology, 119(4), pp. 465–482. Available at: < https://doi.org/10.3764/aja.119.4.0465 >
Sempere, E., 1982. Rutas a los alfares: España-Portugal. Barcelona: E. Sempere.
Seseña, N., 1997. Cacharrería popular: la alfarería de basto en España. Alianza Editorial.
Silvestrelli, F., 2016. Ceramic Production at Sant’Angelo Vecchio and in the Metapontine Chora. In: I.E.M. Edlund-Berry and F. Silvestrelli ed. 2016. The Chora of Metaponto 6: A Greek Settlement at Sant’Angelo Vecchio. University of Texas Press, pp. 129–142.
Silvestrelli, F. and Edlund-Berry, I.E.M., 2016. The Chora of Metaponto 6 : A Greek Settlement at Sant’Angelo Vecchio. Austin: University of Texas Press.
Tomei, F., 2017. Fornaci greche e magnogreche di VIII-VI secolo a.C. Scuola di Specializzazione in Beni Archeologici. Universita’ degli Studi di Padova.
Tomei, F., 2022a. Ancient Greek pottery workshops in their rural landscape setting. Exploring the intersection between production, environment, society and agrarian economy. Available at: < https://doi.org/10.17638/03169006 >
Tomei, F., 2022b. Classical and Hellenistic pottery kilns from Greek rural areas in their natural and human landscap., In: A. Brysbaert, I. Vikatou, and J. Pakkanen ed. 2022. Shaping Cultural Landscapes. Connecting Agriculture, Crafts, Construction, Transport, and Resilience Strategies. Leiden: Sidestone Press, pp. 241–254.
Wendrich, W., 2013. Archaeology and Apprenticeship : Body Knowledge, Identity, and Communities of Practice. University of Arizona Press. Available at: < http://ebookcentral.proquest.com/lib/liverpool/detail.action?docID=3411… > [Accessed 02 January 2023]