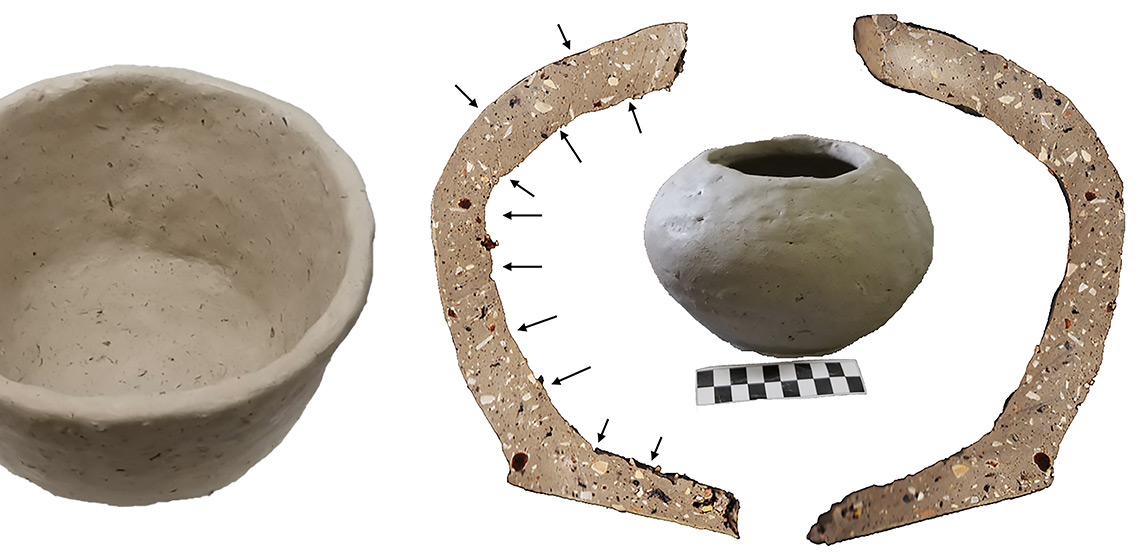
This contribution presents the results of experiments using a simple but effective inclusion and void orientation method for identifying shaping techniques on cut and scanned vessels and sherds. Not only does it provide an additional line of complementary evidence for differentiating ceramic chaînes opératoires, but we argue that it offers observations not accessible by other imaging methods and scales of analysis.
Introduction
Pottery constitutes a major component of the archaeological record and materializes many dimensions of economic and social life. The description of production sequences (chaînes opératoires) provides the empirical basis for comparing and contrasting technical practice and the underlying knowledge required to undertake them. A study of tools, materials, gestures, and the organisation of operations (or techniques) involved in technical practice describes alternative ways of ‘making’ and ‘doing’ and thus identifies the ‘social perimeters’ of shared knowledge distinctive of a particular social network/community/group (Haudricourt, 1987; Lemonnier, 1992; Naji and Douny, 2009; Roux, 2014, p.103). A major focus in the archaeological study of ceramics is to further develop techno-stylistic analysis by improving identifications of shaping techniques on sherd assemblages, with a view to delineating distinct communities of practice in the archaeological record.
With this in the background, experimental studies on vessel shaping have mostly concentrated on visual inspection of surface features (macrotraces) and radiography (internal structure) to clarify and improve the diagnostics of shaping techniques and potting methods on replica vessels and sherd assemblages (e.g. Berg, 2008; 2011; Berg and Ambers 2014, pp.549, 558; Doherty, 2015; Jeffra, 2013; 2019, p.9; Quinn, 2013, p.176). Due to the narrow limits on the field of view, a concern over vessel shaping remains a ‘fringe’ interest in thin-section microscopy and is usually subsidiary to characterising the clay and inclusions before confirming general macroscopic observations at the microscale (Menelaou, et al., 2016, p.487; Quinn, 2013, pp.174-181; Roux, 2011; 2014; Santacreu, 2014, pp.77-79; Thér, 2016, 2020, p.3). A well-known and major limiting factor in the analysis of vessel shaping is the random preservation of surface features found on what is usually a very small minority of pottery fragments relative to the recovered assemblage (Quinn, 2013, p.176; Thér, 2020, p.1). These marks are typically distorted or eradicated by subsequent steps in the production sequence, thus introducing an element of ambiguity. Thér highlights two potential major sources of misinformation: when features are “correlated with a particular technique but are not necessarily a consequence of this technique” and when features “are a necessary consequence of a particular technique but could also be a consequence of another technique” (Thér, 2020, p.1). The complexity of the shaping process and the considerable scope for variability makes this stage in the chaîne opératoire particularly difficult to unravel for archaeological assemblages (Fowler, 2014a; Gosselain, 1998; 2000; 2010). It is for these reasons that the shaping stage is arguably the most poorly understood stage in the manufacture and is even ‘missing’ in many studies on ceramic technology (Jeffra, 2019, p.3; Mentesana et al., 2015, p.4; Roux, 2014).
This paper reports on a simple but effective imaging method trialled on experimental vessels using an ordinary desktop scanner at the Ceramic Technology Laboratory (CTL), University of Manitoba (Winnipeg, Canada). Scans of radial plain-oriented pottery sections were prepared from experimental bowls to test whether known shaping techniques have identifiable and diagnostic features. We have previously published on this alternative imaging method in recent papers on the Early Bronze Age Tell eṣ-Ṣâfi/Gath ceramic assemblage (Ross et al., 2018; 2020). The site overlooks the main east-west pass through the Elah valley of modern Israel where the southern coastal plain meets the lower foothills of the Shephelah (about halfway between Jerusalem and the coast). Tell eṣ-Ṣâfi/Gath was excavated for 25 years resulting in extensive exposures on the Bronze and early Iron Age levels (Maeir 2012; Maeir and Uziel 2020). Shaping techniques were successfully identified on an incredible 82% of the original study sample. However, the effectiveness of this imaging method has yet to be explored and demonstrated using experimental data on vessels made according to a range of different chaînes opératoires. The aims of this preliminary experimental study are therefore threefold: 1) to investigate an alternative perspective on internal vessel structure to further clarify and expand criteria for recognising shaping techniques on sectioned vessel walls; 2) show congruency with existing imaging methods that are carried out at different scales of observation in comparable experimental studies on vessel shaping; and 3) provide some indication of how different clay recipes impact the visibility of the preferred orientation of inclusions at this scale of observation.
The scope of this experimental study is potentially vast. There are countless ways to shape vessels using a variety of different techniques in combination, and there are countless ways to make and prepare suitable clay recipes with different textural characteristics. We, therefore, limited this experimental pilot study to the forming techniques and recipes shown in Table 1 to provide a suitable starting point for a larger ongoing experimental program. We hope to encourage others to add to this data to test and expand on these experimental standards for comparative reference. In this study, we primarily focused on different combinations of coiling, slab, and mould techniques given the large scope for variability extant within these broad categories reported in the ethnographic literature (and the likely possibility of additional unreported techniques and combinations for which no present-day analogue is attested in the published ethnoceramic literature). Various wheel-shaping techniques have been intensively studied according to a well-established set of parameters, and we, therefore, aim to redress somewhat the imbalance for other categories of shaping techniques based on discontinuous pressures (e.g. Baldi and Roux, 2016; Doherty, 2015; Jeffra, 2013; Roux and Corbetta, 1989; Roux and Courty, 1995; 1998; Roux, 2003a; 2009; Roux and Jeffra, 2015;).
Materials and method
The identification of shaping techniques
Thér’s overview (2020) provides a detailed summary of the classification of vessel shaping techniques and the various imaging methods carried out at different scales of observation (the macro, meso, and micro) to recognise these techniques on archaeological samples. The preferred orientation of features in the ceramic body (particle distribution of the coarse and fine groundmass, voids, and inclusions) is the seminal diagnostic for identifying shaping techniques on finished vessels (Berg, 2014, pp.546-7; Carr, 1990; Quinn, 2013, p.176; Rye, 1977, 1981, pp.58-89; Santacreu, 2014, pp.77-78; Thér, 2020; Vandiver, 1988, pp.142-143). Different shaping techniques decompress the clay in different ways and will cause the constituent components to take up a preferred orientation in response to the direction and intensity of specific compressive and/or shear stresses associated with particular potting methods. These deformation/decompression patterns have been repeatedly described and confirmed usually using radiographic data in both qualitative and quantitative experimental and ethnographic studies on vessel structure (Berg, 2008; 2011; 2014, pp.547-9; Pierret, et al., 1996; Rye, 1977; 1981; Thér, 2020). A smaller number of studies report some success at the microscale using thin-section microscopy and even scanning electron microscopy (Philpotts and Wilson, 1994; Quinn, 2013, pp.176-181; Roux and Courty, 1995; Thér, 2016; Whitbread, 1996).
Imaging sections of cut wall profiles
We are certainly not the first to experiment and realise the potential of scanned sections of freshly cut vessels for recognising shaping techniques. Early work by Glanzman (1983) and Vandiver (1988, pp.151-153) provided a glimpse of the rich potential offered by the image capture of substantial sections of cut wall profiles (‘thick sections’). In these instances, cross-sections of vessels were imaged using radiography varying the object position to scan alternate faces and capture joins. The distribution and orientation of inclusions and voids provided strong indicators for the inference of forming techniques and the manufacture sequence. As cameras evolved, more recent studies innovated with high-resolution SLR photography of ceramic breaks and polished sections to identify shaping techniques on substantial rim and body sherds. Livingstone Smith’s doctoral thesis (2007) is particularly innovative in that regard. He combined high-resolution photography of ceramic breaks with radiography to reveal traces of shaping on vessels from West Africa (mainly Cameroon, Togo, and Burkina Faso). Despite the success achieved by imaging plain sections of wall profiles, such studies are few, and the method is rarely utilised to its full potential. This perspective of vessel structure is arguably overshadowed and somewhat neglected by more mainstream macro- and micro- approaches and material science techniques that are now well-published in the field of archaeological ceramic analysis and archaeometry in general (Berg, 2014; Greene, et al., 2017; Laneri, 2011; Roux, 2011; 2014; 2016; Santacreu, 2014, pp.77-79). Not only does it provide an additional line of complementary evidence, but we argue that it offers observations not accessible by other imaging methods and scales of analysis (Kingery, 1987, pp.91-99).
The experimental bowls
A total of 30 shallow and deep bowls 5 to 10 cm high were fashioned according to six chaînes opératoires (5 vessels per chaîne opératoire) using the following shaping techniques: coiling, slabs pressed into a concave mould, slabs pressed onto a convex mould, pinching, and drawing from a clay lump. Combinations of these techniques were also investigated, and different clay temper combinations were tested to see how the size, shape, and density of the inclusions influence the visibility of deformation/decompression patterns. The specific tempering strategies and chaînes opératoires (CO) are described in Table 1.
Commercial clay was used to make the vessels. The advantage of using commercial clay is that it is entirely homogenous and has no tempering material, allowing us to control the frequency and characteristics of the non-plastic additives. As an alternative to the clay of controlled quality, we also experimented with river clay collected from the Assiniboine River in two of the recipes (CO5-6). This glacial till clay was extremely ‘sticky’ and fluid. A mortar and pestle were used to crush ingredients for the temper, and digital weighing scales were used to control the clay to temper ratios for each of the recipes. We did not exceed 25% temper. Although liquid and plastic limits vary for different clays, experimental studies have generally shown that recipes exceeding 30% temper “confer no technical advantage to potters” and decreases vessel strength (Fowler et al., 2019, pp.24-25; 2017; Harry, 2010, p.21). Strength tests by Kilikoglou et al. (1995) experimented on calcareous clay briquettes (15% CaO) with different quantities of coarse quartz temper. They concluded that 20% was the optimal volume “above which no further increase in toughness is found” (Kilikoglou et al., 1995, p.2964). Bones were cleaned (soaked), fragmented, heated (to remove residual water), and crushed to make suitable temper for the requisite recipes (CO1 and CO5). We deliberately chose bird bone (small hollow bones with minimal fat) to contrast with pig bone (large, dense, and very fatty bones) to observe differential effects on the preferred orientation of inclusions and the workability of the clay. For the same reasons, we selected chalk (a soft low-density rock) to contrast with heavier and more angular mineral inclusions, including grog (crushed sherds), limestone, and a variety of large grits. Chalky calcareous clays (marls and rendzinas) are also a very common type of clay experienced and exploited worldwide. For recipes that required sieving (CO1), a 3.5 mm aperture sieve was used to remove the very coarse fraction.
All joints between assembled elements on all pots were smoothed to create the desired preform (with one exception to compare differences in wall morphology). The vessels were built base first and were not fired. They were left to dry in a well-ventilated space in the upright position for one month away from direct sunlight. Contrary to some concerns raised in the ethnoceramic literature (London, 2014), shaping flat bases (using potting methods based on discontinuous pressures) was not a particularly difficult or problematic exercise and did not result in a high failure rate when left to dry upright (also see Ali, 2015; Fowler, 2014b). Potters in eSwatini (borders Mozambique in southern Africa) simply set their finished vessels on the base and the weight of the vessel deforms the rounded base flat (Fowler, 2014b). The experimental bowls were cut and scanned in the bone-dry state at CTL.
CO no. | Recipe (raw materials and processing techniques) | Shaping techniques | Drying time | Surface Treatment | Comment on bowl shape |
1 | Commercial clay. Temper: crushed chicken bone, crushed chalk, sand, grit, and coarse fibre. Wet clay to temper ratio was 20:3 (15%). Temper was sieved and mixed by folding and wedging. | Spiral coiled (continuous) from base to rim. | 1 month | Thoroughly smoothed with the fingers. | Shallow and deep bowls with rounded walls |
2 | Commercial clay. Temper: sand, fibre, large grits and grog. Wet clay to temper ratio was 4:1 (20%). Not sieved and mixed by folding and wedging. Very coarse. | Pressed slabs/sheets of clay joined in a concave mould/vessel support with fabric used as a release agent. Upper body and rim finished off with coiling. | 1 month | Thoroughly smoothed with the fingers. | Deep bowl with straight walls |
3 | Same as no. 2 | Base and lower body formed by pinching and finished off with coiling. | 1 month | Thoroughly smoothed with damp fabric. | Shallow conical bowl |
4 | Same as no. 2 | Pinched out from a clay mass. | 1 month | Burnished with fat using a pebble. | Shallow bowl with rounded /incurving walls |
5 | Commercial clay. Temper: crushed pig bone. Wet clay to temper ratio was 4:1 (20%). Not sieved and mixed by folding and wedging. | Pressed slabs/sheets of clay joined over a convex mould with fabric used as a release agent. Upper body and rim finished off with coiling. | 1 month | Not smoothed (left as a rough-out). | Deep bowl with straight walls |
6 | River clay (Assiniboine). Temper: grits and sand. Wet clay to temper ratio was 4:1 (20%). Not sieved and mixed by folding and wedging. | Ball of clay opened up with the fist and drawn up from a single clay mass. | 1 month | Smoothed with the fingers. | Shallow and deep bowls with straight walls (cylindrical) |
Table 1. The chaînes opératoires (COs) of the experimental bowls
Imaging protocol
Our mesoscopic protocol experiments with a number of simple improvements for imaging trace evidence of vessel shaping on cut vessels and sherds (detailed in Ross, 2020; Ross et al., 2018; 2020). High-quality digital images of ceramic sections are achieved using a conventional flatbed desktop scanner on oriented sherds that are first carefully cut using a wet precision saw to create radial sections. These radial sections expose a flat vertical cross-section of the wall profile. The use of a wet precision saw at relatively low speeds creates a fresh, clean cut. The water acts as a lubricant on a thin 1 mm diamond blade and fragmentation is further minimised by cushioning sherds in a grip/chuck. Dry cuts score and scratch the surface blurring the visibility of features in the section and thicker blades are more likely to fracture delicate sherds. We found that the wet precision saw eliminated the need to use abrasives to polish out scratches in the finished section.
The sectioned vessels and sherds are loaded face down onto the flatbed scanner with a scale. Multiple samples can be scanned at once in batches to speed up the process. We scan one side of the section while it is still damp, since the moisture draws out the contrast between subtle features in the wall profile; the same technique is widely used to photograph and illustrate stratigraphic profiles. Dpi/Scan settings are partly determined by the size of the sampled assemblage, the digital storage capacity available for the project, and the specifications of the laptop/computer. Ordinary printer/scanner combinations are now capable of scanning at high resolution, upwards of 2,000 dpi, which we found was more than sufficient. We did not typically exceed 1,200 to 1,500 dpi per image file. Scanning at higher resolution enables greater magnification at (and even beyond) the equivalent magnification of a handheld lens, hence why we class this method as ‘mesoscopic’. Increased dpi also enables the ability to select and highlight features in the clay body with greater ease when using various selection tools available on photo editing programs. It is possible to achieve microscopic levels of observation and image analysis with more expensive high resolution flatbed scanners. These are used in sedimentary petrography to aid in the characterisation of pores, grains, and particles of rock samples on prepared thin-sections (Van Den Berg, 2003). Application on archaeological assemblages is limited but has shown great promise “for distinguishing and characterising tempers in ceramic materials” with a resolution surpassing 5 μm/pixel (Reedy et al., 2014, p.256).
We use Photoshop to enhance and annotate images because all actions are stored on separate layers and are easily reversible. This final step is optional and potentially time-consuming, but it makes a substantial difference to image quality and the visibility of deformation/decompression patterns in the fabric (optimising image settings, specialised filters, use of tints, reverse negative, greyscale, and so forth). Digital radiography of ceramic objects can involve comparable image enhancement (Berg, 2014, p.553). It is certainly worthwhile annotating some sections using the line tool to visualise the preferred orientation of voids and inclusions. Many observations and notations can be added once the digital image is captured and this only requires basic familiarity with layer-based image editing software (Photoshop, Pixelmator Pro, Image J, et cetera). The binder can be eliminated, and the orientations of inclusions and voids can be highlighted. A host of measurement software programs (e.g. Image J) are available and allow one to quickly extract measurable 2D point data from any image if an accurate scale was included in the original scan. We can measure angles and linear distances on fissures, voids, wall thickness, joints, and other features exposed in the scanned section. For example, by laying a protractor over the scanned image base, it is simple and easy to measure variability in angles and evaluate the regularity of wall construction. This led to the recognition of mould manufacture for some of the vessels in the EBIII assemblage from Tell eṣ-Ṣâfi/Gath in the absence of large numbers of whole forms (Ross et al., 2020). The main limitation is time at this point, since it can become a highly labour intensive process when manually carried out on every image. Clearly there is potential to automate this process with machine learning to pick out deformation/compression patterns and some promising progress has already been made in that direction at CTL (Koval, 2018).
Results
Chaîne opératoire 1: spiral coiling (See Figures 1-3)
Orientation distributions: Coiling techniques were clearly recognised on all samples and all form elements (rim, body, and base). The long axes of non-plastics were aligned in a radial direction or followed the spiralling folds of a coil. As shown in Figure 2, some coils consistently have more temper than others despite fairly intensive mixing. Individual coils can be picked out by the varied quantities and texture of the coarse inclusions. The distributions of non-plastics form wavy, undulating, and spiral patterns in section. Open spaces dominated by binder have edges bordered and encircled by clusters of coarse non-plastics creating discontinuities at regular intervals in the fabric and thus marking the location of coil joints. In these open spaces, non-plastics tend to be smaller in size and sparse in quantity. This pattern appeared with strong regularity across all sections in CO1.
Wall morphology: The preferred orientation of features in section are congruent with irregularities in ‘bumpy’ wall profiles from the deformation of individual coils. When seams/joints were preserved on vessel surfaces they did correspond with the preferred orientation of fabric features exposed in the scanned section. This was more noticeable on the interior of deeper vessels with a more restricted orifice (See Figure 3). These features were identified with greater ease on the scanned sections, especially when magnified on high-resolution colour images. Despite intensive smoothing with the fingers, walls still preserved the outline (however faint) of deformed coils, often marked by mm differences in wall thickness. To the naked eye, these variations are almost imperceptible, but are easily recognised (and measured) on scanned sections. Across the area of the spiral coiled base, wall thickness increases incrementally with distance from the midpoint of the base (See Figure 3.). In contrast, flattened bases that are rolled/stretched out tend to be thinner towards the edge of the base and thicker at the midpoint (i.e. the reverse of what is seen here).
Chaîne opératoire 2: slab construction in a concave mould with coiled rim (See Figures 4-6)
Orientation distributions: Shaping techniques were not easily identifiable based on the orientation and distribution of non-plastics. The decompression patterns were generally weak. Nevertheless, the majority of inclusions in the mould made parts of the body were aligned subparallel to vessel walls and are therefore consistent with the pattern/orientation produced by simple compression forces when the clay slabs/sheets were flattened. There is a noticeable difference with the orientation and distribution of inclusions seen in the area of the rim (above the red line on Figure 4), and these patterns are consistent with the coiling technique discussed in C01. The joints between slabs are not easily visible but are sometimes marked by significantly larger inclusions and a slight reduction in wall thickness. This is most likely due to the extra pressure applied to seal and work the joined elements and can cooccur with fissures perpendicular to the vessel wall (See Figure 5; Vandiver, 1988, p.143).
Wall morphology: The base was highly symmetrical in contrast with bowls made freehand (See Figure 6). Walls were far more uniform and standardised within and between samples in CO2 and contrast significantly with the ‘bumpy’ morphology seen in CO1. The only exception was the area of the rim, which was finished off with coils and has a more corrugated profile with noticeable inflection points. The coiled parts of the vessel walls are noticeably thicker than the slab/mould made parts of the body, and this was still recognisable despite intensive smoothing operations (compare Figures 4-6 with Figure 9). Walls increase in thickness and are less regular/uniform above the lip of the mould. In this zone, coil deformations are more visible on the exterior surface (the surface in contact with the mould). Figure 9 shows the wall morphology when coils were not finished with smoothing operations to complete the preform.
Chaîne opératoire 3: pinched pot with coiled rim (See Figure 7)
Orientation Distributions: The visibility of the orientation and distribution of fabric features was similar to CO2, which is not wholly surprising given that the recipes were identical. The decompression patterns were weak, and it was not easy to isolate the shaping techniques solely based on the characteristics of the non-plastics. However, the coarse inclusions did snake in S-like patterns where the vessel was finished off with coiling, but the pattern was not particularly strong across all sections. No joints or clear discontinuities in the fabric were identified in the lower part of the body. This fits our expectation, given that this part of the vessel was pinched out from a single clay mass. Compression forces and pressures were therefore relatively weak/mild compared to other shaping techniques that strongly deform and reconfigure the internal structure (such as wheel throwing and coiling techniques). The majority of the inclusions in the pinched body had a more random arrangement and were weakly oriented subparallel to vessel walls.
Wall morphology: Wall profiles lacked regularity and were highly variable in thickness and shape (within and between sections from the same vessel), especially compared to CO3. The walls were highly uneven. The thickness of the small flat 5 cm wide base also varied substantially (See Figure 7). Slight dips and depressions from finger impressions were visible on vertical wall surfaces despite intensive smoothing operations.
Chaîne opératoire 4: pinched pot (See Figure 8)
Orientation distributions: The orientation and distribution of fabric features was similar to CO3 except that there was no trace of coiling deformation with weak orientation sub-parallel to vessel walls. Larger inclusions tended to sit and cluster in areas where there was a corner point or strong inflection point (particularly where the lower body connects with the flat base).
Wall morphology: Compared to CO3, walls were far more uniform, regular, and thinner, but were not as uniform as CO2. Wall surfaces still carried dips and depressions from fingertips where the clay was pinched and squeezed to shape the small palm-sized bowl (despite intensive surface treatment, including burnishing techniques). The walls had a curvy profile and not a straight profile. Slight inflections seem to mirror the dimensions of human fingers and thumbs.
Chaîne opératoire 5: slab construction on a convex mould with a coiled rim (See Figure 9)
Orientation distributions: No clear pattern is apparent from the sections, and these samples were arguably the most ambiguous. Coarser inclusions were generally subparallel to vessel walls in the mould-made body, and some inclusions exhibited a very weak spiral patterning consistent with coiling in the area of the rim (although not as apparent as CO1-3). Large coarse inclusions were oriented perpendicular to walls only in the area of the coiled rim (usually at the joint between coils). In a small minority of cases, circular cavities were located at the midpoints of relic coils.
Wall morphology: These sections were more prone to breakage, especially at the point where the first coil was affixed to the slab. The walls were not as even and regular as other vessels made with the aid of a vessel support. Walls were especially uneven in the area of the rim where vessels were finished with coiling.
Chaîne opératoire 6: opened with the fist and drawn up freehand (See Figure 10)
Orientation Distributions: All inclusions point in the same direction the clay was pulled and stretched out by the drawing technique (horizontal across the base and vertical in the walls). There are no discontinuities or joints visible in the clay body since the vessel was formed from a single clay mass. Inclusions are moderately well sorted compared to the poorly sorted inclusions typical of coiled vessels.
Wall morphology: Walls are thick and straight(ish) with smooth surfaces that taper from the edge of the base with some irregularity in wall angles. There are no dips, depressions, or corrugations.
CO no. | Workability of the clay | Preferred orientation of non-plastics | Visibility of Compression pattern | Wall morphology | Figures |
1 | High | Radial directions, or follow the spiralling folds of a coil. Wavy, undulating and spiral patterns. Poorly sorted coarse particles cluster on joints | Strong | Irregular and bumpy walls with variations in thickness from deformed coils. Thick rounded walls. | Figs 1-3 |
2 | Low | Sub-parallel to vessel walls in the body. Same as CO1 in the area of the rims. Sorting is more uniform across the body compared to the rim. Joints between slabs are often interrupted by very coarse inclusions. | Weak | Reduced variation in wall thickness with greater regularity in wall profiles across the body. Corrugated rims with bulges more prominent on the exterior surface. Thin straight walls (deep). | Figs 4-6 |
3 | Low | Weakly oriented subparallel to vessel walls across the body with weak wavy and spiral s-shaped patterns in the area of the rim. | Weak | Significant variations in wall thickness. No regularity/consistency in wall thickness. Thick flaring walls | Fig 7 |
4 | Low | Coarse inclusions cluster at corner and inflection points and are subparallel to vessel walls. | Weak | Uniform walls with slight inflection points, indentations, and dips from fingertips. Incurving thin walls (shallow). | Fig 8 |
5 | Extremely poor | Coarse inclusions are limited to subparallel alignments in the body but vary across the area of the rim. In a minority of instances, circular voids occur in the centre of coils. | No pattern | Same morphology as CO2 with reduced regularity | Fig 9 |
6 | Medium (verging on too sticky) | Coarse inclusions point in the direction the clay was drawn and stretched (horizontal across the base and vertical across the body). Inclusions are moderately well sorted with no discontinuities in the clay body. | Moderate /strong | Thick straight(ish) walls of varied thickness. Walls taper with distance from the exterior edge of the flat base. | Fig 10 |
Table 2. Summary of results on the preferred orientation of inclusions, visibility of compression pattern, and wall morphology.
Discussion
The size, shape, and mass of the temper had a clear observable impact on the orientation and distribution of fabric features seen in section. Very coarse temper, greater in size than half the diameter of the vessel wall, was difficult to work and did not generate particularly good results in terms of the patterns visible in section (CO2-5, Table 2). This temper also created difficulties when burnishing the surface and resulted in a mottled effect. Very fine amorphous inclusions with no clear preferred orientation were also uninformative. It is well known that these kinds of studies on preferred orientation work best on fabrics with “elongated, lamellar, or prismatic particles” (Santacreu, 2014, p.78). Crushed chalk (CO1) produced great results in terms of both the workability of the clay and the compression patterns visible in section. Bird bone was also an excellent temper (CO1), but not all bone temper was an equal success. Despite intensive cleaning and heat treating prior to use, pig bone was disastrous (CO5). Coils were very greasy, fragile, and disintegrated when heavily manipulated. The fat released by the bone was simply incompatible with the clay (and the high water content), producing a mass that was very difficult and unpleasant to work (The clay body smelt unpleasant and will almost certainly not survive a firing). In addition, the pig bone fragmented differently from the bird bone producing splinters that inflicted injury on the hands. Although fibre was added as temper to CO2-4 (See Figure 5), it was not a particularly salient feature for picking out deformation/decompression patterns relative to other temper types. However, it was not a major component of the recipe, and future studies may wish/should investigate the patterning generated by vegetal/fibre temper on fired pots (the resulting voids will presumably be more distinct in fired sections).
Clay temper combinations with good workability (for shaping techniques using discontinuous pressures) generated far better compression patterns visible in section for differentiating shaping techniques. But even clays with poor workability and more ambiguous deformation/compression patterns still yielded useful information from the imaged sections to aid in the identification of the potting methods. This is good news since past potters were no doubt far better (and more particular) at selecting, processing, and making good clay recipes with desirable properties to make their pots. In many instances, the results were better than expected, given the intensive joining and smoothing operations. Sieved temper (CO1) in well-mixed clays produced the best results, since size ranges and distribution were more consistent. Choice of clay processing techniques clearly impacts the orientation and distribution of inclusions and voids and therefore impacts the identification of shaping techniques.
Despite intensive smoothing operations (including burnishing with a stone for several hours), wall morphology seen in section was a surprisingly helpful index for confirming the identification of shaping techniques (especially when the image is so easily magnified without distortion). Distinct changes in wall profile (Table 2) were observed on sections subject to various combinations of shaping techniques (i.e. slab and coil techniques with and without supports). According to Berg (2014, p.547), highly experienced potters will not achieve ‘perfect cohesion’ for every coil joint. Evidence will survive somewhere on the pot irrespective of the intensity of the smoothing and secondary forming techniques.
Contrary to expectation, void distribution was not a particularly useful criterion when compared to the distribution of non-plastics. We expect this is because sections were cut when bone-dry in an unfired state and were smoothed/polished by the wet saw, which likely diminished the integrity of the void pattern. The void pattern was certainly an important diagnostic in our study of scanned sections from the Tell eṣ-Ṣâfi/Gath EBIII assemblage (Ross et al., 2020). Future experimental studies can improve on our results by firing the experimental replicas and further experimenting with different types of clays and tempers, particularly bodies with sparse inclusions and vegetal tempers. In this study, we primarily focused on recipes with more abundant inclusions.
Conclusions
This experimental study aims to refocus attention on the utility of plain cut sections for identifying potting methods in chaîne opératoire research. The simple scanning method demonstrates the effectiveness of using ordinary desktop flatbed scanners for imaging trace evidence of potting methods on cut wall profiles and refreshed edges of sherds. The scanned sections nicely visualise the different compression patterns and wall morphologies to aid in differentiating the chaînes opératoires of the experimental bowls. Previous studies primarily used some form of photography and/or radiography to visualise features indicative of shaping techniques at greater cost for images with lower resolution. The data capture of raw (unprocessed) image files is rapid and straightforward, but enhancing and annotating scanned images can be time-consuming. Nevertheless, this last step is optional and can be automated with machine learning for large datasets (Koval, 2018).
The differential effects of temper strategies on the preferred orientation of inclusions were indicated for varied types of bone and mineral temper. Our results are by no means comprehensive on this front but provide a good baseline for further exploratory research. Our experimental control group provides useful standards for comparative reference clarifying identification criteria. In fact, the results are better than expected since we found that the recipes with optimal workability were also the recipes that produced the clearest deformation/compression patterns in section. The scanned sections still supplied useful information for recipes with poor workability and poor results where identifications were more ambiguous.
The imaging method is flexible and versatile and can be incorporated into larger research programs that combine micro and macro approaches to clarify the most misunderstood stage in the potting process. The main constraint on the sample is that the cut object has to fit on the flatbed scanner, which does not pose much of a problem for the vast majority of archaeological ceramic assemblages. The deformation/compression patterns discussed in this paper were primarily based on qualitative categories for 2D datasets. Providing a scale was included in the original scan, it is possible to collect large amounts of quantitative data on angles and linear measurements of features in section. The visual image holds more information than can be documented or reported. This was originally pointed out by Freestone (1991, p.400) in relation to ceramic thin-sections, and it certainly holds true for larger ‘thick-sections’. Scanned sections can be imported into simple (and/or complex) measurement software to provide metrics useful for measuring variability and standardisation (e.g. the indices for wall regularity manually measured in Roux, 2003b). Such measurements helped reinforce the identification of moulds and vessel supports on the Early Bronze assemblage from Tell eṣ-Ṣâfi/Gath (Ross 2020; Ross et al., 2020).
Clearly, the major drawback is the highly destructive nature of the analysis. Sherds and parts of vessels are dissected with a wet precision saw to reveal the internal structure. The destructive nature of the imaging protocol will invariably limit access to assemblages and curated collections and will impose limits on sample sizes, especially at sites where pottery is less common and permission is needed from local communities. Scanning the sherds with a micro-CT (Computed Tomography) will show the orientation distributions of inclusions and voids without harming sherds and vessels, with the added advantage of a 3D image (Applbaum and Applbaum, 2005; Kahl and Ramminger, 2012; Takenouchi and Yamahana, 2021). Analysis of the orientation and distribution of inclusions and voids is still very much possible, but at significantly greater cost, expertise, and additional machine time. Alternatively, smaller subsamples of sherds and discards can be cut and sectioned when stricter antiquity laws prevent mass export and/or destructive analysis of large sherd assemblages. At Tell eṣ-Ṣâfi/Gath, the sections of large body sherds and bases were more useful for identifying fashioning techniques than the rims. Rims were usually retained as indicatives for typological studies. The majority of the base and body sherds were therefore available for a technological study involving destructive analyses. Often, however, such sherds are discarded on tell sites in the Near East (even at Tell eṣ-Ṣâfi/Gath) due to the ubiquity of ceramics in this region.
No specialist equipment or laboratory facilities are required. The work can be conducted in any facility that has space to go through the assemblage, with an appropriate area to set up a wet saw, a computer/laptop with photo editing software, and an ordinary flatbed scanner. The actual data acquisition is rapid since large numbers of sherds can be cut and scanned in batches. Scanning takes longer than cutting, but no longer than a few minutes at most (even when scanning at high dpis). Processing the data can be highly time-consuming, depending on the effort invested in generating enhanced annotated images that highlight the trace evidence of vessel shaping. As stated previously, the last step can be automated by machine learning or dropped from the workflow completely. Images do not have to be enhanced and annotated for a visual inspection. We look forward to further developing this useful ‘mesoscopic’ scanning method in future studies. We hope that others will similarly benefit from this scanning method and mesoscopic perspective of cut vessel walls. Our proposed protocol is intended to supplement existing approaches and not replace them, enabling multiscalar and multivariate research programs to boost identifications of pottery forming practices on archaeological datasets.
Acknowledgements
A special thanks goes to Tiffany Okaluk and her potting skills for assisting the authors and making some of the great pots for this experimental study (specifically CO1, CO2, and CO4). We also thank two anonymous reviewers for feedback and suggestions on improvements to the paper. Any errors are the responsibility of the authors.
Bibliography
Ali, N., 2015. The Human Aspect of Technology: An Ethnoarchaeological Study of Cooking Ware From Jordan. Near Eastern Archaeology, 78, pp.80-87.
Applbaum, N. and Applbaum, Y., 2005. The use of medical computed tomography (CT) imaging in the study of ceramic and clay archaeological artifacts from the ancient Near East. In M. Uda, G. Demortier, and I. Nakai, eds. 2005. X-rays for archaeology. Dordrecht: Springer, pp.231-245.
Baldi, J. and Roux, V., 2016. The innovation of the potter's wheel: A comparative perspective between Mesopotamia and the southern Levant. Levant, 48, pp.236-253.
Berg, I., 2008. Looking through pots: Recent advances in ceramics x-radiography. Journal of Archaeological Science, 35, pp.1177-1188.
Berg, I., 2011. Exploring the chaîne opératoire of ceramics through x-radiography. In S. Scarcella, ed. 2011. Archaeological ceramics: A review of current research. Oxford: Archaeopress, pp.57-63.
Berg, I. and Ambers, J., 2014. X-radiography of archaeological ceramics. In A. Hunt, ed. 2014. The Oxford handbook of archaeological ceramic analysis. Oxford: University Press, pp.544-564.
Carr, C., 1990. Advances in ceramic radiography and analysis: Applications and potentials. Journal of Archaeological Science, 17, pp.13-34.
Doherty, S. K., 2015. Origins and use of the potter's wheel in ancient Egypt, Oxford: Archaeopress.
Fowler, K., 2014a. Ethnography. In A. Hunt, ed. 2014. The Oxford handbook of archaeological ceramic analysis. Oxford: University Press, pp.469-486.
Fowler, K., 2014b. Ceramic production in Swaziland. Anthropos, 109, pp.411-428.
Fowler, K., Basco, K. and Fayek, M., 2017. Pre-contact pottery tempering practices at Sipiwesk Lake, Manitoba: The effects of grit tempering on drying and firing. Canadian Journal of Archaeology, 41, pp.97-116.
Fowler, K., Shabaga B., Brownlee, K. and Fayek, M., 2019. Identifying precontact ceramic resource areas in the boreal forest of northern Manitoba, Canada. North American Archaeologist, 40, pp.3-35.
Freestone, I. C., 1991. Extending ceramic petrology. In A. Middleton and I. Freestone, ed. 1991. Recent developments in ceramic petrology. British Museum Occasional Paper. London: British Museum Press, pp.399-410.
Glanzman, W., 1983. Examination of xeroradiographic pottery manufacturing techniques: A test case from the Baqah Valley, Jordan. Masca Journal, 2, pp.163-169.
Gosselain, O., 1998. Social and technical identity in a clay crystal ball. In M. T. Stark, ed. 1998. The archaeology of social boundaries. Washington: Smithsonian Institution Press, pp.78-106.
Gosselain, O., 2000. Materializing identities: An African perspective. Journal of Archaeological Method and Theory, 7, pp.187-217.
Gosselain, O., 2010. Exploring the dynamics of African pottery cultures. In B. Randi, A. Engevik, and I. Oye, ed. 2010. The archaeology of regional technologies: Case studies from the Paleolithic to the age of the Vikings. New York: The Edwin Mellen Press, pp.193-224.
Greene, A. F., Hartley, C. W., Doumani Dupuy, P. N. and Chinander, M., 2017. The digital radiography of archaeological pottery: Program and protocols for the analysis of production. Journal of Archaeological Science, 78, pp.120-133.
Harry, K., 2010. Understanding ceramic manufacturing technology: The role of experimental archaeology. In J. Ferguson, ed. 2010. Designing experimental research in archaeology: Examining technology through production and use. Boulder: University Press of Colorado, pp.13-46.
Haudricourt, A. G., 1987. La technologie science humaine. Recherches d’histoire et d’ethnologie des techniques. Paris: Editions de la Maison des Sciences de l’Homme.
Jeffra, C., 2013. A re-examination of early wheel potting in Crete. The Annual of the British School at Athens, 108, pp.31-49.
Jeffra, C., 2019. CRAFTER: Potting techniques of the Bronze Age. EXARC 2019/1, pp.1-14.
Kahl, W.A. and Ramminger, B., 2012. Non-destructive fabric analysis of prehistoric pottery using high-resolution x-ray microtomography: A pilot study on the Late Mesolithic to Neolithic site Hamburg-Boberg. Journal of Archaeological Science, 39, pp.2206-2219.
Kilikoglou, V., Vekinis, G. and Maniatis, Y., 1995. Toughening of ceramic earthenwares by quartz inclusions: An ancient art revisited. Acta Metallurgica et Materialia, 43 (8), pp.2959-2965.
Kingery, D. W., 1987. Microstructure analysis as part of a holistic interpretation of ceramic art and archaeological artifacts. Archeomaterials, 1, pp.91-99.
Koval, O., 2018. Using supervised machine learning to classify ceramic fabrics. Unpublished M.A. Thesis, Department of Anthropology, University of Manitoba.
Laneri, N., 2011. The life-history of the potter's wheel in the ancient Near East. In S. Scarcella, ed. 2011. Archaeological ceramics: A review of current research. Oxford: Archaeopress, pp.64-72.
Lemonnier, P., 1992. Elements for an anthropology of technology. Michigan: Museum of Anthropology, University of Michigan.
Livingstone Smith, A., 2007. Chaîne opératoire de la poterie: Références ethnographiques, analyses et reconstitution. Tervuren: Musée royal de l’Afrique centrale.
London, G., 2014. Appendix ID: Manufacturing technique of late third millennium BCE flat-bottomed pots. In W. G. Dever, ed. 2014. Excavation at the Early Bronze IV sites of Jebel Qa'aqir and Be'er Resisim. Winona Lake: Eisenbrauns, pp.253-277.
Maeir, A. M., 2012. Tell eṣ-Ṣâfi/Gath I. Report on the 1996–2005 seasons. Ägypten und Altes Testament 69. Wiesbaden: Harrassowitz.
Maeir, A. M. and Uziel, J., 2020. Tell eṣ-Ṣâfi/Gath II. Excavations and Studies. Ägypten und Altes Testament 105. Münster: Zaphon.
Menelaou, S., Kouka, O. and Day, P., 2016. Pottery production and exchange at the Heraion, Samos during the late third millennium BC: First steps in the study of technology and provenance. Journal of Archaeological Science Reports, 7, pp.480-488.
Mentesana, R., Day, P. M., Kilikoglou, V. and Todaro, S., 2015. United in our differences: The production and consumption of pottery at EM IB Phaistos, Crete. Journal of Archaeological Science: Reports, 7, pp.489-498.
Naji, M. and Douny, L., 2009. Editorial. Journal of Material Culture, 14, pp.411-432.
Philpotts, A. R. and Wilson, N., 1994. Application of petrofabric and phase equilibria analysis to the study of a potsherd. Journal of Archaeological Science, 21, pp.607-618.
Pierret, A., Moran, C. J. and Bresson, L. M., 1996. Calibration and visualization of wall-thickness and porosity distributions of ceramics using x-radiography and image processing. Journal of Archaeological Science, 23, pp.419-428.
Quinn, P. S., 2013. Ceramic petrography: The interpretation of archaeological pottery & related artefacts in thin section, Oxford: Archaeopress.
Reedy, C. L., Anderson, J., Reedy, T. J. and Liu, Y., 2014. Image analysis in quantitative particle studies of archaeological ceramic thin sections. Advances in Archaeological Praxis, 2(4), pp.252-268.
Roux, V. and Corbetta, D., 1989. The potter's wheel: Craft specialization and technical competence, New Delhi: IBH Pub. Co.
Roux, V. and Courty, M. A., 1995. Identification of wheel throwing on the basis of ceramic surface features and microfabrics. Journal of Archaeological Science, 22, pp.17-50.
Roux, V. and Courty, M. A., 1998. Identification of wheel-fashioning methods: Technological analysis of 4th-3rd Millennium BC oriental ceramics. Journal of Archaeological Science, 25, pp.747-763.
Roux, V., 2003a. A dynamic systems framework for studying technological change: Application to the emergence of the potter's wheel in the southern Levant. Journal of Archaeological Method and Theory, 10, pp.1-30.
Roux, V., 2003b. Ceramic standardization and intensity of production: Quantifying degrees of specialization. American Antiquity, 68, pp.768-782.
Roux, V., 2009. Wheel fashioned ceramic production during the third millennium BCE in the southern Levant: A perspective from Tel Yarmuth. In S. A. Rosen and V. Roux, ed. 2009. Techniques and people: Anthropological perspectives on technology in the archaeology of the proto-historic and early historic periods in the southern Levant. Paris: de Boccard.
Roux, V., 2011. Anthropological interpretation of ceramic assemblages: Foundations and implementations of technological analysis. BAR International Series, 2193, pp.80-88.
Roux, V., 2014. Ceramic manufacture: The chaîne opératoire approach. In A. Hunt, ed. 2014. The Oxford handbook of archaeological ceramic analysis. Oxford: University Press, pp.101-113.
Roux, V. and Jeffra, C., 2015. The spreading of the potter's wheel in the ancient Mediterranean: A social context-dependent phenomenon. In W. Gauss, G. Klebinder-Gauss, and C. Von Ruden, ed. 2015. The transmission of technical knowledge in the production of ancient Mediterranean pottery. Austria: Österreichisches Archäologisches Institut, pp.165-182.
Roux, V., 2016. Ceramics and society: A technological approach to archaeological assemblages, Cham: Springer International Publishing.
Ross, J., Fowler, K. D., Shai, I., Greenfield, H. J. and Maeir, A. M., 2018. A scanning method for the identification of pottery forming techniques at the mesoscopic scale: A pilot study in the manufacture of Early Bronze Age III holemouth jars and platters from Tell eṣ-Ṣâfi/Gath. Journal of Archaeological Science Reports, 18, pp.551-561.
Ross, J., 2020. What makes a pot: Transformations in ceramic manufacture and potting communities in early urban societies of the 3rd millennium bce – a chaîne opératoire perspective from Tell eṣ-Ṣâfi/Gath (Israel). Unpublished PhD Thesis, Department of Anthropology, University of Manitoba.
Ross, J., Greenfield, H. J., Fowler, K. D. and Maeir, A. M. 2020. In search of Early Bronze Age potters at Tell eṣ-Ṣâfi/Gath: A new perspective on vessel manufacture for discriminating chaînes opératoires. Archaeology Review Cambridge, 35, pp.74-89.
Rye, O. S., 1977. Pottery manufacturing techniques: X-ray studies. Archaeometry, 19, pp.205-211.
Rye, O. S., 1981. Pottery technology: Principles and reconstruction, Washington: Taraxacum.
Santacreu, D. A., 2014. Materiality, techniques and society in pottery production: The technological study of archaeological ceramics through paste analysis, Warsaw/Berlin: De Gruyter Open Ltd.
Takenouchi, K. and Yamahana, K., 2021. Fine pottery shaping techniques in Predynastic Egypt: A pilot study on non-destructive analysis using an X-Ray CT scanning system. Journal of Archaeological Science: Reports 37, pp.1-16.
Thér, R., 2016. Identification of pottery-forming techniques using quantitative analysis of the orientation of inclusions and voids in thin sections. Archaeometry, 58, pp.1-17.
Thér, R., 2020. Ceramic technology. How to reconstruct and describe pottery-forming practices. Archaeological and Anthropological Sciences, 12, pp.1-22.
Van Den Berg, E. H., Bense, V. F. and Schlager, W., 2003. Assessing textural variation in laminated sands using digital image analysis of thin sections. Journal of Sedimentary Research 73, pp.133-143.
Vandiver, P. B., 1988. The implications of variation in ceramic technology: The forming of Neolithic storage vessels in China and the Near East. Archeomaterials, 2, pp.139-174.
Whitbread, I. K., 1996. Detection and interpretation of preferred orientation in ceramic thin sections. In T. Higgins, P. Hand, and J. Lang, ed. I1996. maging the past: Electronic imaging and computer graphics in museums and archaeology. London: British Museum, pp.173-181.